Analysis of the Healing Efficiency of Self Healing Concrete
Info: 18188 words (73 pages) Dissertation
Published: 23rd Jun 2021
Tagged: ConstructionCivil Engineering
Abstract
This research intended to analyse the crack-healing efficiency of autonomously self-healing concrete developed through the incorporation of bacteria and an organic medium into the cement matrix as a means of inducing MICP. It also intended to assess the effects incorporating these healing agents into the concrete, through their encapsulation in lightweight aggregates, has on concrete strength and serviceability. Developing on research conducted at the University of Bath (Alazhari, M., 2017), ratios of 3:7 and 4:6 of aggregate impregnated with bacteria (Bacillus Pseudofirmus) to aggregate impregnated with an organic medium (calcium acetate and yeast extract) were added to a standard concrete mix as a 20% or 50% replacement of the mass of sand. A number of prisms were made for each of these mixes, a standard mix and control mixes. These prisms were cured, a crack of approximately 0.1mm wide was induced in each of them and then they were placed in an environmental chamber suited to promote the activity of bacteria to encourage healing. The extent of the healing of the concrete was assessed through the use of ISAT tests and visual analysis under an optical microscope, whilst compressive and flexural strength tests provided an indication of the effects different quantities of lightweight aggregate had on concrete strength. Strength test results showed a reduction in concrete flexural strength with an increase in the quantity of perlite in a mix. Alternatively, the increase of perlite did not share the same effect on compressive strength. Prisms of 50% sand replacement were of considerably low compressive strength, however prisms of 20% sand replacement containing the healing agents necessary for MICP exhibited a similar compressive strength to that of standard concrete, whilst the control mix of the same level of sand replacement displayed a lower compressive strength. ISAT results and visual analysis indicated a similar pattern, with mixes of 50% sand replacement achieving the least satisfactory results with only one mix of 50% sand replacement exhibiting any healing. Standard and control mixes all experienced a degree of autogenous healing, however mixes of 20% sand replacement including both bacteria and medium-containing perlite showed greater healing than any standard or control mixes. Prisms of the mix with 20% replacement of the mass of sand with perlite at a ratio of 3:7 exhibited the greatest level of healing. Ultimately, results indicate that there is a limit to the level of lightweight aggregate that can be added to a standard concrete mix before the beneficial effects of healing cease to be effective and strength begins to deteriorate. They also confirm that MICP is a more effective method of self-healing than relying solely on autogenous healing, whilst the most effective ratio of perlite containing bacteria to perlite containing organic medium is 3:7. This facilitates the determination of an ideal direct ratio of bacteria to organic medium and therefore the ability to assess the reliability of quantities of healing agents used in existing research and for the implementation of more effective tests in future research work.Table of Contents
Abstract.................................................................
Acknowledgements.........................................................
Table of Contents..........................................................
List of Figures.............................................................
List of Tables.............................................................
List of Symbols and Abbreviations.............................................
1.0 Introduction..........................................................
1.1 The Problem
1.2 Criterion for Self-healing Concrete
1.3 Basis of Research
1.4 Research Objectives
2.0 Literature Review......................................................
2.1 Methods of Producing Self-healing Concrete.......................................
2.2 Selection of Healing Agent Components..........................................
2.2.1 Bacteria.................................................................
2.2.2 Medium.................................................................
2.3 Incorporation of Bacteria and Medium into Concrete.................................
2.3.1 Encapsulation in Lightweight Aggregates......................................
2.3.2 Compressed Powders and Microcapsules......................................
2.4 Quantities and Ratios of Medium to Bacteria.......................................
2.4.1 Differences in Quantities within Existing Research...............................
2.5 Applications of Self-healing Concrete.............................................
2.6 Summary...................................................................
3.0 Experiments..........................................................
3.1 Achieving Objectives..........................................................
3.1.1 Objective 1..............................................................
3.1.2 Objective 2..............................................................
3.2 Mix Designs.................................................................
3.3 Methodology................................................................
4.0 Results and Discussions................................................
4.1 Initial Crack Widths...........................................................
4.2 Concrete Strength Tests.......................................................
4.2.1 Results.................................................................
4.2.2 Discussion
4.2.2.1 Porosity and the Effects of Voids.....................................25
4.2.2.2 Proportions of Constituent Concrete Elements...........................26
4.2.2.3 Effects of Bacteria on Strength.......................................28
4.2.2.4 Effects of Aggregate Coating and Strength.............................29
4.2.3 Summary................................................................
4.3 ISAT Tests..................................................................
4.3.1 Results.................................................................
4.3.2 Discussion
4.3.2.1 Effects of Excessive Perlite Content.................................31
4.3.2.2 Autogenous Healing.............................................31
4.3.2.3 MICP Healing...................................................32
4.3.2.4 Identification of Ideal Ratio of Healing Agents.........................32
4.3.3 Summary................................................................
4.4 Serviceability................................................................
5.0 Conclusions..........................................................
6.0 Limitations...........................................................
6.1 Time Constraints.............................................................
6.2 Adherence to British Standards..................................................
6.3 Issues Collecting Pre-healing Data...............................................
References...............................................................
List of Figures
Figure 1.1 | Graphical illustration of the aggregate content of concrete mixes used in experiments conducted at the University of Bath |
Figure 1.2 | Healing in concrete prisms achieved during experiments conducted at the University of Bath |
Figure 2.1 | Graph depicting the effect direct incorporation of different constituent elements of MICP healing has on the compressive strength of concrete |
Figure 2.2 | Lightweight aggregate containing bacteria and medium |
Figure 2.3 | Healing in concrete prisms achieved during experiments conducted at the University of Bath |
Figure 2.4 | Water seepage through cracks in concrete made in samples of different cracking ages |
Figure 3.1 | Modified cap used for ISAT tests – (a) Section across length of cap, (b) section across width of cap, (c) plan view of cap, (d) isotropic view of cap |
Figure 3.2 | ISAT Test apparatus |
Figure 3.3 | Plastic location plates for COD gauge |
Figure 3.4 | COD gauge and three point bending apparatus |
Figure 3.5 | Environmental Chamber |
Figure 4.1 | Representation of concrete surface finish (a) Standard mix – STD, (b) 20% perlite replacement of the mass of sand – C20, (c) 50% perlite replacement of the mass of sand – C50 |
Figure 4.2 | Representation of aggregate content (a) Standard mix – STD, (b) 20% perlite replacement of the mass of sand – C20, (c) 50% perlite replacement of the mass of sand – C50 |
Figure 4.3 | ISAT Test Results |
Figure 4.4 | Autogenous healing in prism STD_B |
Figure 4.5 | Autogenous healing in prism C20_B |
Figure 4.6 | Autogenous healing in prism 20-PB3_B |
Figure 4.7 | MICP healing in prism 20-PB3_C |
Figure 4.8 | MICP healing in prism 20-PB4_B |
Figure 4.9 | MICP healing in prism 20-PB4_C |
Figure 4.10 | Autogenous healing in prism C20_B |
List of Tables
Table 3.1 | Concrete mix designs for test prisms |
Table 4.1 | Initial crack widths in concrete test prisms |
Table 4.2 | Compressive and flexural strength test results |
Table 4.3 | ISAT Test Results |
Table 4.4 | Ratio of each healing agents in mix 20-PB3 |
List of Symbols and Abbreviations
MICP | Microbiologically-induced calcite precipitation |
ISAT | Initial surface absorption test |
COD | Crack opening displacement |
PB | Perlite containing Bacteria (Bacillus Pseudofirmus) |
PN | Perlite containing Nutrients (Calcium acetate and yeast extract) |
STD | Standard concrete mix as described in BS EN 196-1-2016 |
C20 | Control mix – Adaptation of STD, with 20% mass of sand replaced with equivalent mass of PN |
C50 | Control mix – Adaptation of STD, with 50% mass of sand replaced with equivalent mass of PN |
20-PB3 | Adaptation of mix STD, containing impregnated perlite PB and PN in the ratio of 3:7 as a 20% replacement of the mass of sand |
20-PB4 | Adaptation of mix STD, containing impregnated perlite PB and PN in the ratio of 4:6 as a 20% replacement of the mass of sand |
50-PB3 | Adaptation of mix STD, containing impregnated perlite PB and PN in the ratio of 3:7 as a 50% replacement of the mass of sand |
50-PB4 | Adaptation of mix STD, containing impregnated perlite PB and PN in the ratio of 4:6 as a 50% replacement of the mass of sand |
Ff | Flexural failure load of concrete during (kN) |
Fc | Compressive failure load of concrete (kN) |
Rc | Compressive strength of concrete (kN) |
Rf | Flexural strength of concrete (kN) |
l | Length of prism (mm) |
b | Width of prism (mm) |
Vagg | Volume of aggregate (cm3) |
magg | Mass of aggregate (kg) |
ρagg | Density of aggregate (kg/m3) |
Vperlite | Volume of perlite (cm3) |
Vsand | Volume of sand (cm3) |
CSACT | Cross-sectional area of a capillary tube (m2) |
LCT | Length moved along the capillary tube (mm) |
CSAcap | Wetted-contact area of cap (m2) |
1.0 Introduction
This dissertation aims to investigate the crack-healing efficiency of MICP as a means of developing an effective self-healing concrete. The primary objectives are to assess the healing capabilities of different ratios of the healing agents required for MICP and the effects of their incorporation into concrete via the impregnation of lightweight aggregates on concrete’s mechanical properties. To achieve these objectives, a series of tests were conducted on a number of different concrete mixes and the results have been compared. Ultimately, this dissertation will contribute to wider research aiming to improve concrete maintenance and reduce refurbishment costs for concrete structures. Most excitingly, however, by incorporating living organisms into concrete structures, this work can bring the built environment to life.1.1 The Problem
Concrete’s versatility, durability and exceptional compressive strength have made it the most prevalent construction material in the world and the second-most used substance in the world after water (Al-Tabbaa, 2017). Despite this popularity, it suffers from one major flaw; its comparatively low tensile strength leaves it susceptible to the formation of cracks. Sub-millimeter micro-cracks increase the permeability of concrete, allowing the ingress of waterborne chemicals and contaminants that will corrode steel rebar and attack the cement matrix. Consequently, maintenance and repair of structures costs the EU approximately €6 billion per year (Spinks, 2015), whilst engineers are forced to overdesign the steel reinforcement in concrete structures in order to resist crack formation (Delft University of Technology, 2016). It has therefore become of interest to the civil engineering sector to develop an autonomously self-healing concrete, limiting the requirement for human intervention in its repair thereby reducing maintenance and construction costs and extending the service life of structures. Research has shown that such a concrete can be achieved through the use of MICP. This incorporates the use of bacteria, an organic precursor and nutrients within the cement matrix; the bacteria have the ability to use the nutrients and precursor provided to secrete calcium carbonate (calcite), a healing compound that is used to fill the cracks that form in concrete.1.2 Criterion for Self-healing Concrete
The majority of existing research on developing self-healing concrete, particularly that concerned with MICP, largely focuses on a small number of influential factors in the healing process. Research, including this dissertation, is guided by parameters that the constituent elements of MICP must conform to in order to maximise healing efficiency and minimise detrimental effects to the structural and aesthetic properties of concrete. These include the assurance that the healing agents do not have any adverse effects on the concrete, that the concrete does not have any adverse effects on the healing agents, that the most efficient healing agents for the promotion of calcite precipitation are used and that the process is renewable and sustainable (Paine, 2016).1.3 Basis of Research
Recently, researchers at the University of Bath have investigated the effect different ratios of bacteria to organic medium have on the crack-healing efficiency of MICP (Alazhari, M., 2017). One of the intentions of the research was to determine an ideal ratio of bacteria to medium. A substantial amount of existing research uses extremely different ratios of bacteria to medium, or does not state the quantities used at all; obtaining evidence of an ideal ratio of bacteria to medium would facilitate more accurate interpretations of existing research and would have drastic implications for research that fails to evidence their mix proportions accurately. During these experiments, bacteria (Bacillus Pseudofirmus) and organic medium (calcium acetate and yeast extract) were encapsulated separately in perlite then incorporated into a standard concrete mix at a variety of ratios as a 20% replacement of the volume of standard sand, as illustrated by fig. 1.1. Fig. 1.1 – Graphical illustration of the aggregate content of concrete mixes used in experiments conducted at the University of Bath
A set of concrete prisms was made for each mix design. Once given adequate time to cure, the prisms were cracked and the healing of these cracks was assessed over time through the use of ISAT tests and detailed visual analysis under a microscope. Comparison of ISAT test results and visual inspections, both before and after allowing the prisms time to heal, provided a quantitative analysis of the extent of healing in each prism, and therefore a comparison of the healing capabilities of each mix. Fig. 1.2 – Healing in concrete prisms achieved during experiments conducted at the University of Bath – Bottom left of each image shows ratios of PB:PN, (Alazhari M., 2017)
Results of these experiments indicated that the most efficient ratios of PB to PN were 4:6 and 3:7, as evidenced by fig. 1.2. The standard concrete control mix shows no signs of healing, in contrast to the mixes containing PB to PN at ratios of 4:6 and 3:7; the white deposits shown in the images are deposits of calcite secreted by the bacteria to fill the cracks. It is therefore important to conduct further testing to validate these results and analyse the two most successful ratios of PB:PN (3:7 and 4:6) in greater detail. Once these results have been verified with further tests, an ideal direct ratio of bacteria to medium can be calculated.
1.4 Research Objectives
This dissertation intends to develop these experiments conducted at the University of Bath by conducting further tests on the ratios 3:7 and 4:6 of PB to PN in MICP self-healing concrete.Objective 1: | Further assess the crack-healing efficiency of the ratios 3:7 and 4:6 of PB to PN in MICP self-healing concrete |
Objective 2: | Determine the extent to which using lightweight aggregates as a vehicle for the incorporation of bacteria and nutrients into concrete affects concrete strength and serviceability |
2.0 Literature Review
This review provides a summary of existing research that investigates the use of MICP as a means of self-healing concrete, delivering critical analysis of contemporary literature. Despite the fact that this concept is still in its infancy, there has been extensive research conducted by experts throughout the industry analysing the identification of the most appropriate constituent elements, the methods of their incorporation into concrete, alternative methods of achieving a self-healing concrete and the current and potential applications of self-healing concrete in construction. Importantly, it identifies a significant flaw in existing research; a lack of uniformity in the quantities and ratios of bacteria to organic medium used in different self-healing concrete research projects, and often the absence of any evidence of the quantities and ratios used.2.1 Methods of Producing Self-healing Concrete
There have been a number of alternative methods explored in recent years for creating a self-healing concrete. Ghent University (2014) conducted a number of studies investigating the incorporation of super-absorbent polymers into the cement matrix that expand when in contact with water. In practice, these expanded polymers will plug cracks that develop in the concrete; nonetheless the cracks are only truly ‘repaired’ after the manual application of solid healing products. This, therefore, is not a successful means of drastically reducing maintenance costs and developing a ‘healing’ concrete, despite having limited success at protecting the cement matrix and rebar. Investigations conducted by Wang and De Belie (2016) on the enzymatic hydrolysis of urea produced promising results in physically healing cracks through the precipitation of calcite, as opposed to simply plugging them. However, the vast quantities of ammonia released during this process generated excessive atmospheric nitrogen loading, a cause for considerable environmental concern. Subsequently, it has been necessary to conduct detailed exploration into alternative methods of producing an efficient self-healing concrete. One of the most promising methods of achieving such a concrete is through the use of MICP. This method incorporates the use of alkaliphilic bacteria and organic medium within the cement matrix; the bacteria have the ability to use the medium to secrete calcite (limestone), the compound used to fill cracks generated in the concrete. In contrast to the enzymatic hydrolysis of urea, research has found that metabolic conversion of calcium salts has very few environmental repercussions. Although the immediate reaction of the calcium salt with oxygen emits carbon dioxide, it has been suggested by Jonkers, et al. (2016) that this is a two-stage process, the second stage of which is homologous to carbonation: Initial Reaction:2.2 Selection of Healing Agent Components
Research indicates that bacteria used in MICP must have the capacity to withstand the aggressive environment within concrete, particularly during hydration; this includes a resistance to high compressive forces exerted by reduction in pore diameter size during hydration (Jonkers, et al., 2010) and, to a lesser extent, resistance to high alkaline pH (Paine, 2016). It could be argued that high temperature resistance is an unnecessary parameter as cracks form at the concrete surface, where hydration temperatures are not especially high. The presence of the medium and bacteria within the cement matrix must not adversely affect the rate of hydration or mechanical properties of the concrete (Paine, 2016), whilst the two must work efficiently together and respond quickly to the formation of the cracks. Ultimately, the bacteria and medium must have sufficient longevity within the concrete to make MICP a viable method of inducing self-healing in concrete.2.2.1 Bacteria
Research widely advises that germinated and active bacteria are far more susceptible to environmental stresses than bacteria in their spore form. Study by Jonkers, et al. (2010), developed by Seifan, Samani and Berenjian. (2016), has determined that bacteria within the genus Bacillus conformed to the outlined criteria, with the capability to survive at high temperatures and pH afforded to them by their ability to sporulate. If incorporated in the concrete in its spore form can theoretically survive for approximately 200 years under the appropriate conditions (Schlegel, 1993). Bundur, Kirisits and Ferron (2015) conducted experiments showing that direct incorporation of bacteria, specifically S. Pasteuri (a bacteria very similar to those commonly used for self-healing applications, such as Bacillus Pseudofirmus), in a cement paste adversely affected hydration kinetics. The bacteria delayed the onset of hydration and resultantly reduced the early strength of the concrete, despite providing an equivalent degree of strength and hydration at 28 days as a cement mix without the presence of bacteria. Interestingly however, the presence of live versus dead bacteria present in the cement paste was examined to see what effect each had on the concrete. Results indicated that the presence of dead bacterial cells created a weaker mix compared to a mix containing viable and active cells (fig. 2.1). Ultimately this indicates that even though the presence of cells in any state may adversely affect the onset and rate of hydration, active cells may work within the cement matrix to increase concrete strength and thereby compensate for their initial negative impact.
Fig. 2.1 – Graph depicting the effect direct incorporation of different constituent elements of MICP healing has on the compressive strength of concrete, (Bundur, Kirisits and Ferron, 2015)
2.2.2 Medium
Paine (2016) outlines that any nutrients used in this process must conform to two main criteria; they must aid germination of spores and provide sufficient sustenance for growth and development of the bacterial cells. Paine refers to work by Bundur, Kirisits and Ferron (2015) that suggested yeast extract in particular delays the onset and reduces the rate of hydration. Similarly, Jonkers, et al. (2010) found that yeast extract reduced the compressive strength of concrete. Research completed by Jonkers, et al. (2010) specifies that calcium lactate is the only organic precursor capable of direct integration into the cement matrix that will actively increase the compressive strength of the concrete. This research determines that the additions of other calcium salts (e.g. calcium acetate) have a detrimental effect on the mechanical properties of the concrete if added in a similar way. Subsequently, the detrimental effects of certain nutrients, precursors and bacteria on the concrete and vice versa, has driven researchers to explore a variety of different techniques of incorporating them into the cement matrix to negate these issues.2.3 Incorporation of Bacteria and Medium into Concrete
There are a number of different methods for incorporating the bacteria and medium into the concrete mix that have been investigated. These include placing the bacteria and/or medium directly into the mix (often in the form of solution added to mix water), encapsulating the bacteria and/or medium within porous lightweight aggregates and forming compressed powder based healing agents.2.3.1 Encapsulation in Lightweight Aggregates
Paine (2016) outlines the necessity and associated advantages of encapsulation of both bacteria and medium. He states that encapsulation of bacterial spores and medium within a lightweight aggregate prevents them from interfering with the setting of the cement during hydration, whilst reference is made to the theory proposed by Jonkers (2011) that placing un-encapsulated bacterial cells directly into the matrix will cause large compressive forces to be exerted on hydration, reducing the level of viable bacteria available to be used for healing. Therefore, experiments conducted at the University of Bath conducted by Paine, et al. (2016) have encapsulated bacteria and medium, separately, in lightweight aggregates. Separate encapsulation of medium and bacteria minimises the likelihood of early germination of spores and premature interaction between the medium and bacteria which would impact the rate of hydration, mechanical properties of the concrete and also leave less healing agent available for use when it would be required to heal cracks. To prevent early seepage of bacteria and medium into the concrete, aggregate is coated in a non-permeable membrane comprised of layers of sodium silicate and Portland cement (Paine, et al. 2016). The theory is that when a crack forms in the concrete, it will propagate through the weak, lightweight aggregate, releasing the contents that are then dispersed by the water and interact in the presence of oxygen to secrete calcite (fig. 2.2). Fig. 2.2 – Lightweight aggregate containing bacteria and medium
Work conducted by Tziviloglou, et al. (2015) showed that impregnating lightweight aggregate under standard conditions against impregnating them under vacuum conditions generated significant differences in the loading capacity of the lightweight aggregate. Impregnation under vacuum conditions, regardless of the concentration of the precursor solution, increased the compressive strength of the aggregate almost threefold. This would therefore beg the question, is this increase in compressive strength adequate enough to outweigh the expense of using a vacuum to impregnate the aggregate? It also poses the question, does the use of lightweight aggregate impregnated with medium/bacteria under standard conditions weaken the compressive strength of the concrete to too great an extent to make this a viable solution for the incorporation of MICP into concrete? This will be an issue that will be investigated by conducting experiments in which the level of replacement of the mass of sand with perlite (impregnated under standard conditions) in a standard concrete mix will be varied to assess its effects on strength. Jonkers (2011) has produced experimental results that would indicate that if bacteria were not encapsulated prior to its incorporation in the concrete, it would not survive for more than around 2 months within the concrete matrix. It has been hypothesized that this is a result of continuing concrete hydration, which reduces the pore diameters within the concrete, distorting/crushing the bacterial cells. The author reported that encapsulating bacteria increased its viability to in excess of 6 months, although no precise duration of bacteria viability was provided. Nonetheless, within this paper Jonkers does not outline the method used to determine the quantity of bacteria that survived within the concrete. Common methods for extracting bacteria from hydrated concrete to assess the viability of remaining spores for crack healing are quite rudimentary and it is believed that a great deal of bacteria may even be killed during extraction. Consequently, the reliability of this data must be questioned, as we cannot be sure of the accuracy of the results provided. Research by Jonkers, et al. (2010) mentioned in section 2.2.2, refers to the advantages of using un-encapsulated calcium lactate as an organic precursor. Due to the strength benefits associated with the use of calcium lactate, Wiktor and Jonkers’ (2016) experiments use calcium lactate in an encapsulated form. Experiments conducted at the University of Bath have used calcium acetate (Paine, et al. 2016), a more readily available and cost effective organic precursor than calcium lactate, and like Jonkers and Wiktor have also encapsulated this medium. Encapsulation nullifies any effects the medium may have on hydration rates or mechanical properties of the concrete. Consequently, researchers at the University of Bath are arguably more efficient with their choice of medium; organic precursors are encapsulated to reduce their effects on hydration rates and concrete properties, therefore when encapsulated any possible effects the medium could have on these properties are removed. This defeats the purpose of using calcium lactate, as it was selected on its merits of enhancing concrete strength. It suffices to say that it is far more efficient to use a cheaper, more readily available precursor if ones intention is to encapsulate it in lightweight aggregate.
2.3.2 Compressed Powders and Microcapsules
An alternative method of incorporating bacteria and medium into concrete is by using compressed powder based healing agents. The powdered form of bacteria and medium are compressed into very small capsules. Less healing agent is required in terms of total volume when using this method, therefore in theory could be the solution to high strength concrete applications (Jonkers, et al. 2016). Experimental work in this research dissertation will determine the true extent to which adding substantial amounts of impregnated lightweight porous aggregate reduces the compressive strength of the concrete and therefore potentially provide sufficient cause for the experimentation of compressed powder based healing agents. This dissertation should provide researchers at the University of Bath with information to progress with their desires to proceed with the testing of medium and bacteria-containing microcapsules and their ability to produce an effective autonomic self-healing concrete. Jonkers, et al. (2016) conducted experiments to determine the effectiveness of lightweight porous particle based healing agents against compressed powder based healing agents. Data is provided showing water permeability of 2 cracked concrete prisms over time; the prism containing the compressed powder healing agent reduced the water flow rate through it to 0ml/s in approximately 28 days, indicating complete healing, in contrast to the control prism through which water flow remained relatively constant. The intention of the experiments was to compare the two methods; however in the report this data only exists for compressed powder based healing agents and control samples. Accordingly, if this research dissertation is successful and comprehensive enough to allow researchers at the University of Bath to conduct testing of medium and bacteria microcapsules, then data will be provided on crack healing capacity and the effects of the healing agent on the mechanical properties of concrete. The comparison between these techniques can be made and efforts can be concentrated on the most efficient one.2.4 Quantities and Ratios of Medium to Bacteria
The University of Bath has recently commenced research into the effects the ratio of PB to PN has on the efficiency of crack healing (Alazhari M., 2017). Initial results indicate that ratios of 3:7 and 4:6 (PB:PN) were most successful at healing cracks (fig. 2.3).
Fig. 2.3 – Healing in concrete prisms achieved during experiments conducted at the University of Bath – Bottom left of each image shows ratios of PB:PN, (Alazhari M., 2017)
Whilst analysing the experiments conducted at the University of Bath by Alazhari M. (2017), a potential flaw in the theory behind their experiments became apparent. The ability to heal a crack directly depends on the quantity of medium and bacteria available to interact and produce calcite. The availability of these healing components is intrinsically reliant on the amount of aggregate containing bacteria or medium that a crack propagates through, releasing the contents of the aggregate and making them available for interaction. It is therefore feasible to question the validity of all the results achieved thus far concerning the healing capacity of different ratios of PB to PN as we cannot confirm that each crack has propagated through PB and PN at the same ratio to which they have been incorporated in the mix. All results collected heretofore are unquestionably reliant on the even distribution of encapsulated medium and bacteria throughout the mix and the ratio of perlite containing bacteria to perlite containing medium that a crack has propagated through. Zemskov, Jonkers and Vermolen, (2014) compiled a paper outlining a mathematical model that attempts to prove that the theory behind the process of crack healing via MICP using encapsulated medium is mathematically plausible. Although the assumptions behind this mathematical model are correct, it still fails to completely satisfy the issue originally outlined. The model operates on the assumptions that medium is incorporated in the mix by encapsulating it within lightweight aggregate, whilst the bacteria is incorporated directly in the mix by adding bacteria suspended in solution to the cement paste. The model proves that there will be sufficient cracking of lightweight aggregate to release an adequate quantity of medium that will react with the readily available bacteria. Nonetheless, the research conducted at the university functions under the parameters that both medium and bacteria are encapsulated separately in perlite at a number of different ratios. Consequently, this model cannot be used to prove the validity of the obtained results of varying the ratios of PB to PN. The experiments conducted at the university require numerical proof that cracks propagate through the perlite containing separate healing components at a similar ratio to which the perlite containing the different components is added to the mix, however this research goes beyond the scope of this dissertation.
2.4.1 Differences in Quantities within Existing Research
It is particularly important to note that a great deal of the articles and papers studied show a surprising difference in figures and quantities of healing agents and bacteria used, whilst some research papers fail to provide any detailed description of the quantities of healing agents that have been incorporated into the mixes used during experiments. Subsequently, it is difficult to compare the results of different experiments and to determine if results have been manipulated to suit a desired outcome. In research conducted by Wiktor and Jonkers (2016), healing agents were encapsulated in lightweight clay particles. The paper states that after impregnation with organic medium by soaking in a solution containing 80g/l calcium lactate and 1g/l of yeast extract and suspension in a solution of Bacillus Alkalinitrilicus spores, the expanded clay particles contained 6% by weight of calcium lactate and 1.7x105 bacterial spores per gram of clay particles. Alternatively, research by Paine, et al. (2016) indicates that lightweight aggregate was impregnated with organic medium, consisting of yeast extract and calcium acetate, and Bacillus Pseudofirmus separately by soaking in appropriate volumes of solution. This resulted in 0.3g of calcium acetate (30% by weight), 0.03g of yeast extract and approximately 4.1x109 spores per gram of perlite. Direct ratios of organic medium to bacterial spores can be determined for both research papers and these figures are indisputably crucial to the outcome of each set of experiments and ultimately to the success of self-healing concrete. Consequently, the aforementioned research conducted at the University of Bath (Alazhari M., 2017), research that this dissertation develops, intends to provide a degree of clarification of the ideal ratios of bacteria to medium, and exemplify the importance of the detailed reporting of the quantities of each. The determination of the efficiency of different ratios of bacteria to medium within self-healing concrete will help validate the reliability of existing research work and theoretically provide more uniform and universally comparable results in future research work.2.5 Applications of Self-healing Concrete
The success experienced with the development of MICP in concrete has prompted researchers to assess its viability in full site applications. A substantial amount of work and research has been devoted to the development of a system with the ability to heal existing cracks in structures. Sierra-Beltran, Jonkers and Schlangen (2014) dedicated research to developing a mortar-based MICP healing system, which had a great deal of success at maintaining a high compressive strength of the concrete and efficiently healing existing cracks in structures. Jonkers and Wiktor (2016) have also developed a liquid based repair system to be applied to existing cracks; water leakage tests on cracks treated with the repair system showed a significant reduction in leakage over time, whilst the system also had success in reducing scaling of concrete damaged by freeze-thaw. Successful trials with these systems in both lab-based experiments and site applications have lead to the formation of Basilisk Self-Healing Concrete, a sister company to TU Delft. Its intention is to further develop self-healing concrete, mortar repair and liquid repair systems. As discussed, bacteria have the propensity to sporulate and germinate and can theoretically survive in the concrete for approximately 200 years (Schlegel, 1993), however there is a finite amount of nutrients present in the concrete and crack healing will gradually use this up over time. This suggests that the process is not necessarily sustainable over a prolonged period of time when only relying the nutrients initially incorporated into the mix. Consequently, extensive research is being conducted into the incorporation of a capillary network within concrete structures (Paine, 2016). The network will have the ability to replenish the nutrient supply within the concrete after it has been used up. Work conducted by Luo, Qian and Li (2015) indicates that crack healing capacity is reduced with cracking age (fig. 2.4). This is somewhat concerning as it implies that potentially, under site applications, cracks generated under creep or impact loading many years after the construction of the concrete structure would not be healed efficiently. Fig. 2.4 – Water seepage through cracks in concrete made in samples of different cracking ages, Luo, Qian and Li (2015)
Nonetheless, the bacteria used in the experiments was not encapsulated and as other research discussed previously has shown, un-encapsulated bacteria is susceptible to damage during hydration, which may be the reason for such limited crack healing in older concrete during these tests.
2.6 Summary
A review of contemporary research and knowledge surrounding emerging processes of producing self-healing concrete has revealed some missing details that should be clarified to reinforce some existing theories. This research intends to provide clarification of the effects varying the ratios of PB to PN has on the efficiency of self-healing, in turn emphasising the importance of expressing such figures and quantities (i.e. exact quantities of the bacteria and medium) in all reported findings and research involving developments in self-healing concrete. It will also attempt to provide results that demonstrate the effects large quantities of impregnated lightweight aggregate have on concrete strength and concrete healing capacity. Moreover, if this research is comprehensive enough to provide researchers at the University of Bath with sufficient information to derive a relationship between direct quantities of bacteria to medium in concrete, this will enable them to proceed with the development of the application of medium and bacteria-containing microcapsules as a means of incorporating MICP into concrete.3.0 Experiments
3.1 Achieving Objectives
3.1.1 Objective 1
A set of concrete mixes were designed, as shown in Table 3.1, including a standard mix, control mixes and mixes containing perlite as a replacement of 20% or 50% of the mass of sand. These proportions of sand replacement were used to determine the effects of the addition of large quantities of coated, impregnated aggregate. PB and PN were added to the mixes containing perlite at ratios of 4:6 and 3:7 (PB:PN). Sets of prisms were made for each mix design, cracks were generated in them and a series of ISAT tests were performed to determine the permeability of each prism with the presence of cracks. The prisms were then kept in an environmental chamber, suited for the encouragement of bacterial germination and healing activity, for 28 days. After this time, ISAT tests were repeated to reassess the permeability of the concrete after healing. The comparison of the ISAT results, accompanied by analysis of the cracks under an optical microscope, provided an indication of the extent of crack healing in each prism and thereby an indication of the efficiency of each ratio of PB to PN.3.1.2 Objective 2
Prisms of each mix design were subjected to flexural and compressive strength tests, whilst visual inspection and ISAT tests provided a representation of the serviceability of each mix.3.2 Mix Designs
Mix Reference | Perlite content (% by mass of sand) | PB:PN Ratio | Constituents (g) | ||||
Water | Cement (CEM II/B-V 32.5N) | Standard sand | PB | PN | |||
STD | --- | --- | 225 | 450 | 1350 | --- | --- |
C20 | 20 | 0:1 | 225 | 450 | 1080 | --- | 270 |
C50 | 50 | 0:1 | 225 | 450 | 675 | --- | 675 |
20-PB3 | 20 | 3:7 | 225 | 450 | 1080 | 81 | 189 |
20-PB4 | 20 | 4:6 | 225 | 450 | 1080 | 108 | 162 |
50-PB3 | 50 | 3:7 | 225 | 450 | 675 | 203 | 472 |
50-PB4 | 50 | 4:6 | 225 | 450 | 675 | 270 | 405 |
3.3 Methodology
BS 1881-208-1996 was used as a guide for all ISAT tests conducted during this dissertation, however they were conducted with the use of a modified cap. The cap required for ISAT tests described by BS 1881-208-1996 has a contact surface area of 5000mm2. As such, a modified cap capable of fitting a 40x40x160mm prism was required for these experiments to be conducted. Small, specialised caps were constructed using 3mm thick PVC, each with a water contact surface area of 22 x 30mm (fig. 3.1).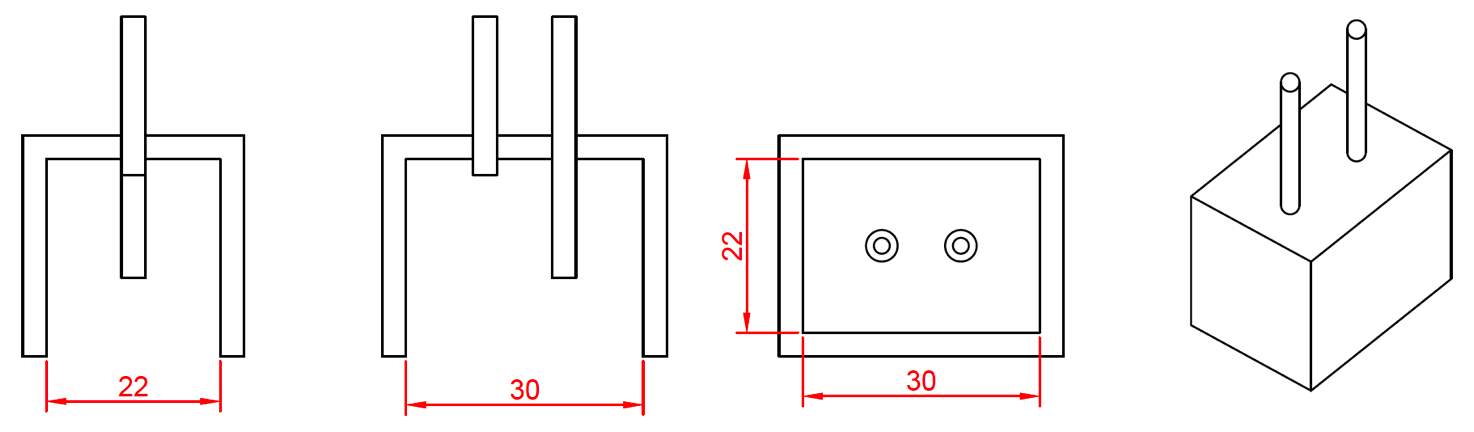
Fig. 3.1 – Modified cap used for ISAT tests – (a) Section across length of cap, (b) section across width of cap, (c) plan view of cap, (d) isotropic view of cap
ISAT tests were used as a method of determining the degree of healing of cracks, rather than for their primary function in assessing the permeability and finish quality of a concrete surface. As such, results were only recorded at the first interval recommended by the standard 10 minutes after the start of the test, as opposed to the full hour long recording period. This provided adequate information to compare pre- and post-healing permeability values to determine the level of healing for each crack. 1. Sets of 3 concrete prisms were made for each mix design, adhering to BS EN 196-1:2016, and a layer of plastic reinforcing mesh was placed at a depth of approx. 1/3 from the bottom of the prism. Prisms were given 24 hours to cure and then removed from their molds. Each set was labeled with its mix reference and given the identity A, B or C within each set. Prism A of each set was to be used for strength tests whilst prisms B and C of each set were to be used for the assessment of the crack healing capacity of each mix design. Two prisms were used for self-healing tests to provide contingency in the event that issues are encountered during testing for one of the prisms from each set, increasing the chances of achieving usable crack-healing data for each mix design. 2. Prism A of each mix was cured for 28 days at standard temperature ((20,0 ± 1,0)°C) in a water bath (as outlined in BS EN 196-1:2016). They were then subjected to flexural and compressive strength tests at 28 days to assess the strength of each mix (see BS EN 196-1:2016). 3. Prisms B and C from each set were cured for 14 days at 50°C in a water bath. A higher curing temperature was used than that advised in BS EN 196-1:2016 in order to accelerate the curing process, maximising time available for crack healing before the hand-in deadline. An increased curing temperature was chosen over the use of a carbonation chamber so as not to reduce the calcium hydroxide available for the secondary stage calcite precipitation, as outlined in section 2.1. Research suggests that the optimal growth temperature range for Bacillus Pseudofirmus is 10-45°C (Stoica, n.d.), however at 50°C the bacteria remain in their spore form, until conditions are appropriate for germination and growth. 4. After 14 days of curing at 50°C, prisms B and C of sets STD, 20C and 50C were dried at 30°C for 48 hours in preparation for ISAT testing. They were then subjected to ISAT tests to determine a standard value of surface permeability for standard mixes, mixes with 20% perlite replacement of sand and mixes with 50% perlite replacement of sand. ISAT test apparatus is shown in fig. 3.2. Fig. 3.2 – ISAT Test apparatus 5.
Prisms B and C of every mix, including those just subjected to ISAT testing, were dried at 30°C for 48 hours in preparation for post-cracking ISAT tests. 6. Grooves were etched across the centre of the bottom face of each prism to encourage crack propagation through this point. Plastic location plates were fixed to each prism above these grooves using araldite – these plates acted as ‘stirrups’ for a COD gauge used to monitor crack size during crack generation. Fig. 3.3 shows the shape of the plates; the two vertical slits fit the prongs of the COD gauge, giving them a separation of 5mm and allowing for a displacement of anywhere up to 3mm. The semicircular features allow the two halves to sit securely together.
Fig. 3.3 – Plastic location plates for COD gauge 7.
Each prism was subjected to three-point bending using a displacement rate of 0.03mm/min to generate a crack of 0.1mm wide along the bottom surface of the prisms, where the reinforcing mesh in the prisms limited the depth of crack propagation. Since research by Jonkers, et al. (2016) suggests that crack widths of up to 0.8mm can be healed, a crack width of 0.1mm was chosen to increase the likelihood of achieving a reasonable degree of self-healing in the short timeframe available to conduct these experiments. Apparatus used during experiments (fig. 3.4) was the same as that specified in BS EN 196-1:2016. Fig. 3.4 – COD gauge and three point bending apparatus
Load was applied until a crack width of 0.2mm was measured, at which point it was immediately removed; continuing load application past the desired 0.1mm crack width was to account for the elastic strain recovery of the plastic reinforcement. 8. ISAT tests were immediately performed once all prisms had been cracked to measure surface permeability of the concrete with the presence of the crack before healing. 9. Prisms were placed in an environmental chamber (fig. 3.5) on a 24-hour wetting-drying cycle (24 hours wet conditions, 24 hours dry conditions), at an ambient temperature of approximately 23°C, providing conditions suited to promote the activity of bacteria. These prisms were left for 28 days to give allow time for the bacteria to germinate and commence the healing process. Fig. 3.5 – Environmental Chamber 10.
After 28 days in the environmental chamber, ISAT tests were repeated on each prism to determine the extent of the healing. 11. Upon the completion of ISAT testing, the cracks in the prisms were analysed under an optical microscope.
4.0 Results and Discussions
4.1 Initial Crack Widths
Table 4.1 shows readings of crack widths from the COD gauge during crack formation in prisms B and C of each prism set after the removal of load and the elastic strain recovery of the plastic mesh reinforcement. All widths are within a reasonable range of the target width of 0.1mm. Table 4.1 – Initial crack widths in concrete test prismsPrism | Crack width (mm) |
STD_B | 0.103 |
STD_C | 0.112 |
C20_B | 0.118 |
C20_C | 0.103 |
C50_B | 0.102 |
C50_C | 0.136 |
20-PB3_B | 0.098 |
20-PB3_C | 0.093 |
20-PB4_B | 0.110 |
20-PB4_C | 0.127 |
50-PB3_B | 0.100 |
50-PB3_C | 0.116 |
50-PB4_B | 0.121 |
50-PB4_C | 0.116 |
4.2 Concrete Strength Tests
4.2.1 Results
Table 4.2 – Compressive and flexural strength test resultsFlexural Strength Test | Compressive Strength Test | ||||
Prism | Load at Failure, Ff (kN) | Rf (MPa) | Load at Failure, Fc (kN) | Rc (MPa) | Mean Rc (MPa) |
STD_A | 3.27 | 7.67 | 36.02 | 22.51 | 24.00 |
40.78 | 25.49 | ||||
C20_A | 2.37 | 5.56 | 27.90 | 17.44 | 16.26 |
24.12 | 15.07 | ||||
C50_A | 1.25 | 2.92 | 14.68 | 9.18 | 7.06 |
7.93 | 4.95 | ||||
20-PB3_A | 2.47 | 5.79 | 35.93 | 22.46 | 23.09 |
37.95 | 23.72 | ||||
20-PB4_A | 2.55 | 5.97 | 40.31 | 25.19 | 26.84 |
45.58 | 28.49 | ||||
50-PB3_A | 1.10 | 2.58 | 16.12 | 10.07 | 10.11 |
16.24 | 10.15 | ||||
50-PB4_A | 1.47 | 3.45 | 19.55 | 12.22 | 12.12 |
19.25 | 12.03 |
4.2.2 Discussion
4.2.2.1 Porosity and the Effects of Voids
Cracks in concrete will propagate in the direction of least resistance; this is one of the principle assumptions this method of MICP relies upon – healing agents are released from their weak encapsulating aggregate as they provide low resistance to crack propagation (Zemskov, Jonkers and Vermolen, 2014). However, in especially porous concrete, cracks will propagate through voids - this may explain the reduction in flexural strength with an increase in perlite content, and the low compressive strength of mixes C50, 50-PB3 and 50-PB4. Visual inspection confirms that the porosity of concrete increases as the perlite content of the mix increases (fig. 4.1). Accordingly, it is expected that concrete with greater perlite percentage replacement of sand will be more susceptible to crack propagation, and therefore failure in compression or flexure, due to the theoretically larger number of voids present within the concrete than the standard concrete mix, STD. This hypothesis is reinforced by the assumption that a 1% increase in the volume of entrained air in concrete causes a 5% reduction in its compressive strength (Mishra, 2017a).![]() |
![]() |
(a) | (b) |
![]() |
|
(c) | |
Fig. 4.1 – Representation of concrete surface finish (a) Standard mix – STD, (b) 20% perlite replacement of the mass of sand – C20, (c) 50% perlite replacement of the mass of sand – C50 |
4.2.2.2 Proportions of Constituent Concrete Elements
An increase in the total volume of aggregate, without a proportional increase in the quantity of cement and water, will result in an insufficient quantity of cement paste to coat the total surface area of the aggregate creating a weak and porous concrete. The overfilling of the voids between the aggregate by the sand and cement paste is important for workability and ultimately durability of the concrete (Collins, 2017). This once again suggests that as the perlite content of the mix increases, the strength of the mix will decrease. Using equation 4.1 below, the increase in the volume of aggregate from that in a standard mix (STD) to the volume present in mixes containing 20% and 50% perlite replacement by mass of sand can be determined: Vagg=maggρagg![]() |
![]() |
(a) | (b) |
![]() |
|
(c) | |
Fig. 4.2 – Representation of aggregate content (a) Standard mix – STD, (b) 20% perlite replacement of the mass of sand – C20, (c) 50% perlite replacement of the mass of sand – C50 |
4.2.2.3 Effects of Bacteria on Strength
As suggested, strength test results imply that bacteria have had no effect on the flexural strength of concrete, whereas the difference in compressive strength between mix C20 and mixes 20-PB3 and 20-PB4 indicates that the presence of bacteria in concrete has had a constructive effect on its compressive strength. All impregnated perlite used in these experiments was encapsulated with layers of sodium silicate and Portland cement. Research, discussed in section 2, argues that any effects the bacteria within PB would have on early-age concrete strength are negated by the encapsulation of the aggregate (Paine, 2016). It is theoretically possible for abrasion during mixing and placing to remove some of the encapsulating layer on the perlite, bringing bacteria into direct contact with the cement matrix. Section 2.2.1 indicates it has been suggested that un-encapsulated, active bacteria present in concrete will increase the strength of the mix (Bundur, Kirisits and Ferron, 2015). However, Jonkers, et al. (2010) suggest that un-encapsulated bacteria would not survive the compressive forces exerted upon them during hydration for more than a few days – ensuring their survival in the harsh environment provided by concrete is the primary reason for their encapsulation. It is possible that in the short time the bacteria remain viable they have the capacity to increase concrete strength (Paine, 2016), yet the difference in compressive strength seen between C20, 20-PB3 and 20-PB4 is substantial given such a short period of viability of the bacteria. Furthermore, research by Bundur, Kirisits and Ferron (2015) suggests that the presence of killed cells in a mix would have a detrimental effect on concrete strength, whist Jonkers, et al. (2010) states that calcium lactate is the only organic precursor that will not adversely affect the strength of concrete. Therefore, removal of the encapsulating layer of PN would release calcium acetate into the mix, theoretically weakening the concrete, whilst the presence of killed cells in the matrix would potentially negate the strengthening effects the cells had during their active period. Ultimately, research indicates that the encapsulated bacteria should have little to no influence on the concrete’s compressive strength unless it had escaped from its encapsulating aggregate, however test results indicate that either the coating on the encapsulating aggregates was ineffective, or that these results were anomalies. Tests should be repeated on mixes STD, C20, 20-PB3 and 20-PB4 to confirm the effects of bacteria on the compressive strength of concrete.4.2.2.4 Effects of Aggregate Coating and Strength
As referenced in section 2.3.1, the perlite is encapsulated using a combination of sodium silicate and Portland cement. Theoretically, the un-hydrated cement contributed by the coated perlite will reduce the water to cement ratio, thereby increasing strength by generating more hydration products and reducing the quantity of voids in the concrete (Mishra, n.d. (b)). The extent of the effects of this increase in the water to cement ratio are questionable as there is not a uniform increase in strength in all mixes containing perlite. Whilst the mixes containing a 50% perlite replacement of the mass of sand are simply too porous for this increase in hydration agents to have any significant impact, an increase in flexural and compressive strength of all mixes containing 20% perlite replacement of sand would be expected. As this is not the case, it is unlikely that the extra cement has had a substantial effect on concrete strength. It is feasible that the uniform reduction in flexural strength and low compressive strength of mixes C50, 50-PB3 and 50-PB4 is simply a result of the increase in weak coarse aggregate content. Perlite is used as a vehicle for healing agents since its low strength prompts cracks to propagate through the aggregate releasing the nutrients and bacteria; a significant proportion of weak material in a mix will ultimately reduce its strength.4.2.3 Summary
Ultimately, results confirm that there is a negative correlation between the quantity of coated, impregnated perlite in a mix and its flexural strength. However, the primary matter of interest in the strength test results is the difference in compressive strength between mixes C20, 20-PB3 and 20-PB. Analysis of the compressive strength results suggest that a moderate (20% by mass) replacement of sand with coated PB and PN improved compressive strength, however excessive replacement (50% by mass) created a porous, weak concrete. This implies that bacteria have managed to influence the strength of the concrete despite its encapsulation within a lightweight aggregate, provided the concrete porosity is not too great. This hypothesis should be confirmed by further testing on prisms of the same mix designs, adhering more strictly to the standard BS EN 196-1:2016 by disregarding results with a variation of greater than 10% from mean values, thereby gaining a more accurate representation of the true strength of these mixes. This research answers the question posed in section 2.3.1, by indicating that the use of lightweight aggregate as a vehicle for the incorporation of healing agents into the cement matrix does adversely effect the strength of concrete should an excessive quantity be used, which in turn advocates the testing of microcapsules as a means of incorporating healing agents into the mix since volume is required to incorporate a similar amount of healing agent in concrete.4.3 ISAT Tests
4.3.1 Results
Table 4.3 – ISAT Test ResultsISAT Value (ml/m2/s) | |||||
Prism | Pre-crack | Post-crack / Pre-heal | Post-crack / Post-heal | % Improvement | |
STD_B | 0.00 | 2.14 | 0.56 | 73.8 | |
STD_C | 0.00 | 2.32 | 1.33 | 42.7 | |
C20_B | 0.00 | 6.51 | 1.92 | 70.5 | |
C20_C | 0.08 | 9.32 | 2.06 | 77.9 | |
C50_B | 0.22 | 11.94 | 12.34 | -3.4 | |
C50_C | - | - | - | - | |
20-PB3_B | - | - | 0.40 | - | |
20-PB3_C | - | 0.95 | 0.06 | 93.6 | |
20-PB4_B | - | - | 1.09 | - | |
20-PB4_C | - | 2.20 | 0.34 | 84.3 | |
50-PB3_B | - | 4.07 | 0.87 | 78.6 | |
50-PB3_C | - | - | 13.76 | - | |
50-PB4_B | - | - | - | - | |
50-PB4_C | - | 11.86 | 14.56 | -22.8 | |
Fig. 4.3 – ISAT Test Results
Table 4.3 and fig. 4.3 show the ISAT values of the prisms measured at different stages of the experiment. Pre-cracking results provide an approximation of the surface permeability of an un-cracked prism to facilitate comparison with the values obtained after healing – the finish on surfaces of prisms with equivalent perlite content were of a similar quality so tests on all prisms prior to cracking was deemed unnecessary. Post-crack/pre-heal values are those recorded immediately after cracking the prisms shortly before placing the prisms in their environmental chamber, whilst post-crack/post-heal values are those measured after 28 days of healing. Some technical issues were experienced during testing. Prisms C50_C and 50-PB4_B were too porous to achieve a set of ISAT results both before and after allowing time to heal; water would not flow into the capillary tube of the ISAT testing apparatus, instead it would seep directly from the cap out of the prisms. The same issue was experienced with prism 50-PB3_C during post-crack/pre-heal tests, however the same problem was not experienced during the post-healing tests.Unfortunately, there was difficulty bonding the cap to prisms 20-PB3_B and 20-PB4_B, consequently the tests failed. After the failure of the first attempt to conduct tests, the prisms were dried out at 30°C for two days and the test was repeated – similar issues were experienced on the second attempt leaving no post-crack/pre-heal data for these prisms. The strict timeframe available to collect and analyse results meant a third attempt was not deemed productive; the use of two prisms for each mix as a contingency provided usable data despite the failure of these two tests. For each test, the length of movement of water along the capillary tube was recorded for an empirically determined length of time (see BS 1881-208-1996), then scaled to equate to the distance moved along the capillary over a period of 60 seconds. ISAT values were then calculated using equation 4.3:
4.3.2 Discussion
4.3.2.1 Effects of Excessive Perlite Content
ISAT results confirm that a 50% replacement of the mass of sand with perlite creates an excessively porous concrete. The inability to obtain results for prisms C50_C and 50-PB4_B coupled with the increase in ISAT results over time in prisms C50_B and 50-PB4_C evidences the substantial porosity of mixes containing a 50% mass replacement of sand with lightweight aggregate. This also indicates that should the porosity of the concrete be too great, MICP healing will be ineffective, which is likely due to the fact the presence of a large number of pores, the majority of which exceed the size of the maximum crack width capable of healing. The extent of the porosity of these mixes is reinforced by the inability to identify a crack of width 0.1mm in these prisms under the optical microscope. It is possible that the lack of healing in the prisms on 50% sand replacement is because an insufficient quantity of healing agents were released from the perlite – cracks will have propagated through voids in the concrete rather than the perlite and as such the healing agents within the perlite will not have been released and made available to participate in crack healing.
4.3.2.2 Autogenous Healing
Autogenous healing, the natural repair of concrete with hydration products that can occur under the absence of tensile stress in a suitably moist environment (Collins, 2017), was anticipated in the prisms. After cracking, no further tensile stresses were applied to the concrete, and the environmental chamber provided a suitable environment for the manifestation of autogenous healing. This can explain the improvement in ISAT values for prisms that do not contain all of the necessary materials required for MICP. The presence of autogenous healing in the prisms is evidenced in figures 4.4 to 4.6; these figures show the repair of cracks with hydration products, rather than the white deposits of calcite that would be expected after MICP. Whilst there is evidence of autogenous healing, the percentage improvement in ISAT results for prisms 20-PB3_C, 20-PB4_C and 50-PB3_B exceed that of any prisms of the standard or control mixes. This confirms that MICP is a successful means of improving the self-healing capacity of concrete.4.3.2.3 MICP Healing
Alternatively, white deposits within the cracks in figures 4.7 to 4.9 show clear evidence of calcite precipitation. Fig. 4.7 shows partial healing in prism 20-PB3_C, however fig. 4.8 and 4.9 show an impressive level of MICP healing in prisms 20-PB4_B and 20-PB4_C. ISAT results would suggest that mix 20-PB3 was the most effective, with prism 20-PB3_C improving its ISAT value by almost 94% becoming 0.06ml/m2/s, comparable to that of an un-cracked surface. Since the crack images are not representative of the entire crack length, aside from the lack of full crack closure visible in fig. 4.7, the crack must almost be entirely healed. The ability to record post-heal ISAT results for prism 50-PB3_C insinuates that there had been enough healing in the prism to reduce its porosity to a level that would allow an ISAT test to be completed, whilst the same cannot be said for prisms of mix 50-PB4. These results would indicate that the most successful ratio of PB to PN for inducing calcite precipitation is 3:7.4.3.2.4 Identification of Ideal Ratio of Healing Agents
This identification 3:7 as the most efficient ratio of PB to PN for the precipitation of calcite facilitates the derivation of the ratio of bacterial spores to medium. Section 3.2 listed the quantities of calcium acetate, yeast extract and Bacillus Pseudofirmus per gram of coated, impregnated perlite and the total mass of PB and PN used in each mix. As such, the total quantity of each healing agent present in mix 20-PB3 can be calculated and is listed in Table 4.4 below. Table 4.4 – Ratio of each healing agents in mix 20-PB3Calcium Acetate (g) | Yeast Extract (g) | Bacillus Pseudofirmus (spores) |
11.34 | 0.95 | 6.6x1010 |
![]() |
![]() |
(a) | (b) |
Fig. 4.4 – Autogenous healing in prism STD_B | |
![]() |
![]() |
(a) | (b) |
Fig. 4.5 – Autogenous healing in prism C20_B | |
![]() |
![]() |
(a) | (b) |
Fig. 4.6 – Autogenous healing in prism 20-PB3_B | |
![]() |
![]() |
(a) | (b) |
Fig. 4.7 – MICP healing in prism 20-PB3_C | |
![]() |
![]() |
(a) | (b) |
Fig. 4.8 – MICP healing in prism 20-PB4_B | |
![]() |
![]() |
(a) | (b) |
Fig. 4.9 – MICP healing in prism 20-PB4_C |
4.3.3 Summary
The results of these tests confirm that MICP is a successful method of creating a self-healing concrete; prisms 20-PB3_C, 20-PB4_C and 50-PB3_B show a greater level of healing than any of the prisms that do not contain the necessary materials to generate MICP, indicating that the presence of both bacteria and nutrients is beneficial to the level of healing achievable in concrete. Mixes STD, C20 and C50 have experienced a reasonable level of autogenous healing, nevertheless the superior improvement in surface permeability, coupled with evidence provided by visual analysis, of mixes 20-PB3, 20-PB4 and 50-PB3 indicates that MICP has improved the level of healing sustained by the concrete. However, most importantly, the results advocate that the most effective ratio of PB to PN is 3:7; the greater improvement in ISAT values between pre and post-healing tests and visual evidence of calcite precipitation in mixes containing PB to PN in the ratio of 3:7 implies that this is the most successful ratio of PB to PN for promoting MICP in the presence of cracks in concrete.4.4 Serviceability
Pre-crack/pre-heal ISAT results would indicate that an increase in perlite content reduces the finish quality of the concrete. 20% perlite replacement of sand has negligible effect with both pre-crack ISAT values as 0.00ml/m2/s and 0.08ml/m2/s , however 50% replacement of sand sees a substantial detrimental effect on the finish quality of the concrete surface. Both ISAT tests and strength tests would indicate that the addition of perlite as a 50% replacement of the mass of sand in a mix creates a weak, unattractive and fundamentally ineffective concrete. Concrete with this level of perlite content exhibited compressive and flexural strengths that were the lowest of all mixes, far too low to be deemed serviceable. The porosity of mixes with an excessively high aggregate content allows substantial water ingress into the concrete, counteracting the intended benefits of creating a self-healing concrete and reduces the effectiveness of any calcite precipitation experienced by the concrete. Alternatively, mixes containing 20% perlite replacement of the mass of sand were workable and easy to place, achieved a satisfactory finish and mixes containing both bacteria and medium demonstrated compressive strength similar to that of standard concrete. Nonetheless, its lower flexural strength will require a greater quantity of steel reinforcement to be used in these mixes, which defeats one of the goals for using self-healing concrete – to reduce the need to use excessive reinforcement. ISAT tests indicated that mixes of 20% perlite content were capable of exhibiting a substantial degree of crack healing. Ultimately, this indicates that there is a limit to the quantity of impregnated lightweight aggregate that can be added to a standard concrete mix before the beneficial healing effects cease to become effective and the concrete strength is reduced.5.0 Conclusions
This dissertation intended to advance work conducted at the University of Bath on the development of autonomously self-healing concrete by identifying the most effective ratio of PB to PN in promoting calcite precipitation in the presence of cracks and to assess the effects of using large quantities of impregnated aggregate on concrete strength and serviceability. A series of flexural and compressive strength tests, ISAT tests and visual analysis using an optical microscope were conducted to achieve these objectives. Results suggest that an excessive increase in the total volume of aggregate, without a proportional increase in the quantity of cement and water, will create a weak and porous concrete. 50% replacement of the mass of sand in a standard mix (BS EN 196-1:2016) with impregnated perlite drastically increases the porosity of the concrete, rendering it extremely weak in both flexure and compression and unable to heal cracks effectively or simply prevent the ingress of water; both counterproductive qualities ultimately rendering it unfit for practical application. Alternatively, 20% mass replacement of sand with impregnated perlite was more successful. Despite a moderate reduction in flexural strength, concrete mixes with this level of sand replacement containing both PB and PN achieved compressive strengths comparable to that of standard concrete, reinforcing previous research suggesting that the presence of bacteria in concrete increases compressive strength. However, this also implies that the sodium silicate and Portland cement coating on the perlite was ineffective. This indicates that there is a limit to the efficiency of the replacement of sand in a standard mix with aggregate. Further similar tests varying the replacement of sand by volume, as opposed to mass, with coated impregnated perlite will facilitate further experimentation with the healing capabilities and strength of different levels of impregnated aggregate replacement of sand whilst maintaining an effective relationship between the quantity of aggregate and hydration products. ISAT tests and visual analysis ratify that both ratios 4:6 and 3:7 of PB to PN are effective at promoting self-healing in concrete. The porosity of all containing perlite as a 50% replacement of the mass of sand, with the exception of mix 50-PB3_C, rendered the effects of any calcite precipitation insignificant on the surface permeability of the concrete with or without the presence of a crack. Mixes containing 20% replacement of the mass of sand with both PB and PN showed more significant levels of healing than mixes only capable of autogenous healing, therefore it can be concluded that MICP is a more effective method of self-healing that solely relying on autogenous healing. Ultimately, it was established that the ratio of PB to PN of 3:7 is the most effective at promoting calcite precipitation in concrete, consequently the information in dissertation facilitates the use of similar ratios of bacteria to medium in future research projects to maximise the efficiency of healing, whilst it enables critical analysis of the effects of the quantities of bacteria and medium on healing rates in existing MICP research and demonstrates the importance of reporting these values to validate research.6.0 Limitations
To ensure the proper interpretation and application of these results, it is important to acknowledge the limitations of this work.6.1 Time Constraints
The time constraints imposed by this dissertation necessitated the acceleration of the curing process for the concrete prisms that were subjected to cracking in order to provide adequate time for healing and the thorough analysis of test results. Ideally, the prisms would have been cured at the standard temperature advised by BS EN 196-1:2016 for 28 days so results were more easily comparable to research that conformed to British Standards. The deadline also limited the extent of data analysed in this dissertation. If more time was available to continue analysing the healing of the prisms, a better indication of the most effective self-healing combination of PB to PN could be attained. Nonetheless, all information gathered throughout this project has been forwarded to PhD students at the University if Bath who will continue the assessment of the healing of the prisms. Conducting ISAT tests on 14 concrete prisms was a time-consuming process, despite not taking data at the full range of intervals outlined in BS 1881-208-1996. Balancing other academic and personal commitments with this research work left insufficient time to conduct more regular ISAT tests for the full range of time advised by British Standards. More frequent testing would have provided sufficient data to determine the mix with the most prompt healing response to crack formation and the mix with the greatest rate of healing. Nonetheless, the results achieved so far still provide an indication of the healing efficiency for all mixes tested. Crack widths were limited to approximately 0.1mm; a width of this size could be healed by autogenous healing alone and is arguably too small for tests concerning MICP. Although results show a greater degree of healing in mixes containing healing agents, the true extent of the healing capabilities of these mixes have possibly not been established. Nonetheless, a greater crack width may not have experienced a substantial degree of healing in the small timeframe available to complete tests.6.2 Adherence to British Standards
The concrete strength tests did not strictly adhere to BS EN 196-1:2016. Due to time restrictions and a lack of available materials, less iterations of both flexural and compressive strength tests were conducted than required by the standard. The accuracy of the strength values can therefore be questioned – this is particularly important considering the unexpected compressive strength results of prisms 20-PB3 and 20-PB4. The strength tests suggest that the presence of bacteria had a beneficial effect on the compressive strength on concrete, despite encapsulation within the perlite. To determine the reliability of these figures and reasons for their elevated strength, it is advised that further testing is conducted on mixes STD, 20-PB3 and 20-PB4 to the number of iterations required by British Standards. ISAT tests also did not fully conform to the standards defined in BS 1881-208-1996. As previously mentioned, ISAT readings were only taken at ten minutes, not at the full number of intervals advised by the standard. The use of a modified cap also affects the ability of the comparison of these results to other experiments that have used the apparatus described by the standard. These experiments were not concerned with the concrete’s ability to achieve a high standard surface permeability; the aim was to assess the reduction in surface permeability after a crack in the concrete surface had been allowed time to heal. The non-conformities in the tests are of little consequence in this respect; provided that any tests conducted in future use similar apparatus to allow the comparison of results with those achieved by these tests.6.3 Issues Collecting Pre-healing Data
Issues with bonding the cap to the surface of some of the prisms during ISAT tests immediately after cracking meant it was not possible to determine the extent of healing for the cracks in these prisms. Without these ‘pre-healing’ readings a percentage improvement in the permeability of the prisms after healing cannot be determined. Fortunately, a degree of contingency was achieved by using two prisms for the assessment of healing in each mix design, ensuring that results were obtained for each mix design. Despite this, the loss of readings remains a loss of quantifiable data that would otherwise have provided additional, numerical representation of the healing in the prisms. Images of the cracks prior to healing would have been beneficial for comparison of crack healing at given locations on each prism. Visual representation of crack closure would provide another means of accurately quantifying the level of healing for each mix. Unfortunately, a lack of access to the required equipment prevented the visual analysis of cracks prior to healing, leaving no images to use to compare healed cracks with.References
Alazhari, M., 2017. The effect of microbiological agents on the efficiency of bio-based repair systems for concrete. PhD thesis. University of Bath, Bath. Al-Tabbaa, A., 2017. Self-healing Concrete for Low-carbon Infrastructure [online] Available from: http://opentranscripts.org/transcript/self-healing-concrete-low-carbon-infrastructure/ [Accessed 17 Apr. 2017]. Bundur, Z., Kirisits, M. and Ferron, R., 2015. Biomineralized cement-based materials: Impact of inoculating vegetative bacterial cells on hydration and strength. Cement and Concrete Research, 67, pp.237-245. Collins, M., n.d.(a). Autogenous healing. [online] Concrete.org.uk. Available from: http://www.concrete.org.uk/fingertips-nuggets.asp?cmd=display&id=651 [Accessed 14 Apr. 2017]. Collins, M., n.d. (b). Importance of aggregate grading. [online] Concrete.org.uk. Available from: http://www.concrete.org.uk/fingertips-nuggets.asp?cmd=display&id=424 [Accessed 12 Apr. 2017]. Delft University of Technology, (2016). Self-healing of Concrete by Bacterial Mineral Precipitation. [online] Available from: http://www.citg.tudelft.nl/en/research/stories-of-science/structural-engineering/self-healing-concrete/ [Accessed 10 Apr. 2017]. Futurism, n.d. Say ‘Goodbye’ to Cracks, Self-Healing Concrete Has Arrived. Available from: https://futurism.com/videos/say-goodbye-to-cracks-self-healing-concrete-has-arrived/ [Accessed 14 Apr. 2017]. Ghent University, 2014. Development of self-healing concrete at the Magnel Laboratory for Concrete Research. Available from: https://www.youtube.com/watch?v=OH2n07WpyU8 [Accessed 21 November 2016] Jonkers, H., Thijssen, A., Muyzer, G., Copuroglu, O. and Schlangen, E., 2010. Application of bacteria as self-healing agent for the development of sustainable concrete. Ecological Engineering, 36(2), pp.230-235. Jonkers, H., 2011. Bacteria-based self-healing concrete. Heron, 56 (1/2), pp.1-12 Jonkers, H.M., Mors, R.M., Sierra-Beltran, L.M.G., Tziviloglou, E., and Wiktor, V.A.C., 2016. Self-healing of cracks in concrete via bacterial aerobic metabolic conversion of organic compounds. In: Wiktor, V., Jonkers, H. and Bertron, A., eds. RILEM Conference on Microorganisms-Cementitious Materials Interactions, 2016-06-23. Paris: RILEM publications S.A.R.L, pp. Luo, M., Qian, C. and Li, R., 2015. Factors affecting crack repairing capacity of bacteria-based self-healing concrete. Construction and Building Materials, 87, pp.1-7. Microbiology Society, 2015. Using bacteria to make self-healing concrete. Available from: https://www.youtube.com/watch?v=H7frDSx9js8 [accessed October 29th, 2016]. Mishra, G. (n.d.(a)). Effect of air entrained concrete on strength of concrete. [online] The Constructor. Available from: https://theconstructor.org/concrete/air-entrained-concrete-strength-effects/8427/ [Accessed 12 Apr. 2017]. Mishra, G. (n.d.(b)). Factors affecting strength of concrete. [online] The Constructor. Available from: https://theconstructor.org/concrete/factors-affecting-strength-of-concrete/6220/ [Accessed 13 Apr. 2017]. Paine, K., 2016. Bacteria-based self-healing concrete: Effects of environment, exposure and crack size. In: Wiktor, V., Jonkers, H. and Bertron, A., eds. RILEM Conference on Microorganisms-Cementitious Materials Interactions, 2016-06-23. Paris: RILEM publications S.A.R.L, pp. 132-146 Paine, K., Alazhari, M., Sharma, T., Cooper, R. and Heath, A., 2016. Design and performance of bacteria-based self-healing Concrete. In: Jones, M. R., Newlands, M. D., Halliday, J. E., Csetenyi, L. J., Zheng, L., McCarthy, M. J. and Dyer, T. D., eds. The 9th International Concrete Conference 2016. Dundee: , pp. 545-554. Schlegel, H., 1993. General microbiology. 7th ed. Cambridge: Cambridge University Press. Seifan, M., Samani, A. and Berenjian, A., 2016. Induced calcium carbonate precipitation using Bacillus species. Applied Microbiology and Biotechnology, 100(23), pp.9895-9906. Sierra-Beltran, M., Jonkers, H. and Schlangen, E., 2014. Characterization of sustainable bio-based mortar for concrete repair. Construction and Building Materials, 67, pp.344-352. Spinks, R. (2015). The self-healing concrete that can fix its own cracks. [online] The Guardian. Available from: https://www.theguardian.com/sustainable-business/2015/jun/29/the-self-healing-concrete-that-can-fix-its-own-cracks [Accessed 10 Apr. 2017]. Stoica, C., n.d. ABIS Encyclopedia - Bacillus Pseudofirmus. [online] Available from: http://www.tgw1916.net/Bacillus/pseudofirmus.html [Accessed 11 Apr. 2017]. Tziviloglou, E., Wiktor, V., Jonkers, H.M., and Schlangen, E., 2015. Preparation and optimization of bio-based and light weight aggregate-based healing agent for application in concrete. In:International Conference on Self-Healing Materials, ICSHM, June 22-24 2015, Durham, USA. Faculty of Civil Engineering & Geosciences, Delft University of Technology, Delft. Extended Abstract. Wang, J., De Belie, N., 2016. Ureolytic bacteria based self-healing concrete. In: Wiktor, V., Jonkers, H. and Bertron, A., eds. RILEM Conference on Microorganisms-Cementitious Materials Interactions, 2016-06-23. Paris: RILEM publications S.A.R.L., pp.103-114 Wiktor, V. and Jonkers, H., 2011. Quantification of crack-healing in novel bacteria-based self-healing concrete. Cement and Concrete Composites, 33(7), pp.763-770. Wiktor, V. and Jonkers, H., 2016. Bacteria-based concrete: from concept to market. Smart Materials and Structures, 25(8), p.084006. Zemskov, S., Jonkers, H. and Vermolen, F., 2014. A mathematical model for bacterial self-healing of cracks in concrete. Journal of Intelligent Material Systems and Structures, 25(1), pp.4-12.Cite This Work
To export a reference to this article please select a referencing stye below:
Related Services
View allRelated Content
All TagsContent relating to: "Civil Engineering"
Civil Engineering is a branch of engineering that focuses on public works and facilities such as roads, bridges, dams, harbours etc. including their design, construction, and maintenance.
Related Articles
DMCA / Removal Request
If you are the original writer of this dissertation and no longer wish to have your work published on the UKDiss.com website then please: