Systems Accident Analysis of Hoegh Osaka Grounding
Info: 12084 words (48 pages) Dissertation
Published: 11th Dec 2019
1 Introduction
1.1 Background
It is a fact that the shipping industry carries out around 90% of the global trade. Since 1970’s the amount of goods carried by the ships have increased gradually and it went up to 8.02 billion tons of goods in 2008 (Anon, 2012). Shipping is considered as safe and economical although it does involve a number of risks such as: social, personal, environmental, to property and to the operation (Chauvin et al., 2013; Martins and Maturana, 2010). In the last few decades, due to the rapid development of technology in ship structures and consistency of the ship navigation system, the accident rate has dropped down to nearly one ship in every 670 in 2010 from about 1 in every 100 ships in 1910 (Chauvin et al., 2013). This contributes towards lesser number of causalities and improved efficiency and productivity of the ships, although shipping safety has drastically improved it is still estimated that when marine accidents do occur, 75-96% are due to human error. It also showed that human error contributes to between 84% and 88% of tanker accidents; 79% of towing vessel groundings; 89% to 96% of collisions; 75% of allisions; and 75% of fires and explosions. Chauvin et al. (2013) states that most of the maritime accidents occur due to decision errors and from the same it can be stated that human errors are the major contributory factor in maritime accidents.1.2 Aims and objectives
The aim of this dissertation is to analyse and compare the results of Hoegh Osaka grounding accident using Human Factors accident analyses methods. As human error is regularly branded as the major contributory factor in most of the accidents, hence this dissertation will analyse the accident using two different systematic accident analysis methods. In section 1 general background regarding human error contribution in the maritime accident is explained. A literature review of accident trends in maritime domain, accident analysis methods and of old (Human error) and new (System error) views will be presented in section 2. Section 3 will analyse the Hoegh Osaka grounding accident using the two chosen accident analysis methods. In section 4, accident analysis of Hoegh Osaka grounding accident and results will be provided after applying the chosen methods. In section 5 results will be discussed and on evaluation, recommendations will be provided accordingly for system safety in the maritime domain to prevent similar accidents in future. Moreover, both the chosen methods will be compared and methodological recommendation for human factors accident analysis will be provided. Section 6 will provide the conclusion for analysis.2 Literature Review
2.1 Accident’s in Maritime Domain
Maritime accident is an undesirable event or sequence of events that can often lead to loss of life or major injury to any person on or off board and it can also result in various types of property damage. For example, at the beginning of the 20th century the very well-known non-wartime maritime calamity, the Titanic disaster happened. At the time of disaster, the Titanic was carrying around 2,200 passenger and crew members aboard of which only 700 people survived the disaster (Titanic and sinks, n.d.). Maritime accidents were much more disastrous and severe during the wartime which lead to more causalities and damage to the property. For example, a German U-boat torpedoed the British RMS Lusitania which sank within 18 minutes during World War 1 and around 1200 victims lost their lives during the incident (The Lusitania Resource, 2016). Similarly, during the World War 2 the German ocean liner Wilhelm Gustloff was taken down by the soviet submarine and it sank within 45 minutes after being hit by three torpedoes. The disaster took 9,343 lives making it the biggest maritime incident of all time (Time.com, 2016).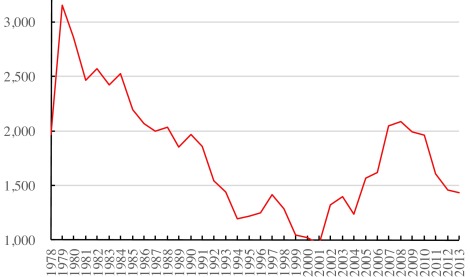
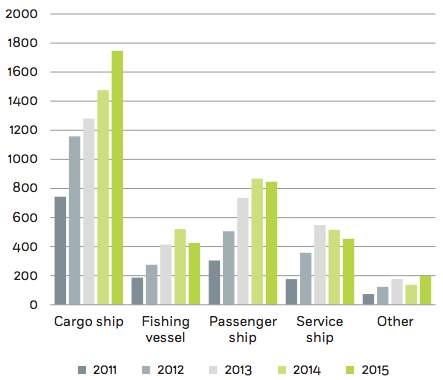
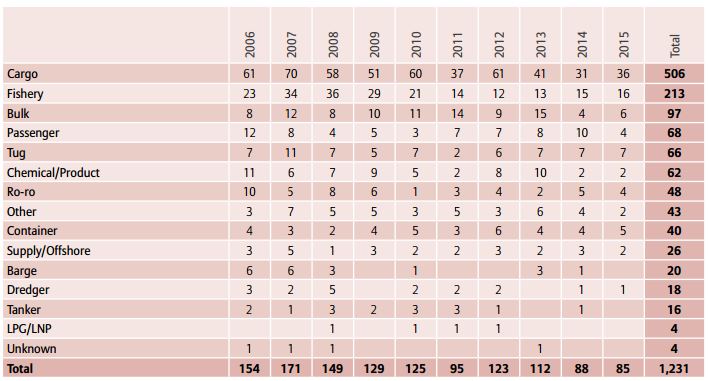
2.2 Human Error
It is constantly cited that 75-96% of accidents occur due to human error (Rothblum, 2002). Hence, this section will explore different types of errors made by humans and these can be identified and classified using error classification. There are many different classification schemes or taxonomies available. However, the most common are the slips and lapse, mistakes and violations classification proposed by Reason (1990). Most common form of human error are slips and they are categorised as errors in which implementation of the required action was improper but purpose or plan was correct. Lapses are hidden error forms that include failure of memory that may not be noticeable in actual behaviour (Reason, 1990). Lapses usually are the failure to perform a planned action or failure to recall the next action required for a sequence. Mistakes are usually present in the unobservable plans and intentions that are planned by an operator. A mistake is a wrong decision or an inappropriate intention followed by the correct execution of the required action (Salmon, Regan and Johnston, 2005). A Violation can be categorised as an intentional or unintentional action that results in incompliance with accepted procedures, standards and rules.2.2.1 Human Errors in Maritime Domain
Human error has been recognised as the main cause in majority of maritime accidents. According to Rothblum (2002) a marine causality study of 100 accidents found that human error directly or indirectly contributed towards 96 of the 100 accidents studied. Of the 96 accidents in 93 accidents multiple human errors were made, by two or more people and every error made was identified as a necessary condition for the incident and if any of the errors could have been prevented then the series of events would not have taken place and the accident would not have happened. There have been many attempts to define the term human error but no collectively accepted definition exists. Rothblum (2002) explains human error as, “an incorrect decision, an improperly performed action, or an improper lack of action” and Reason (1990) described human error as, “a generic term to encompass all those occasions in which a planned sequence of mental or physical activities fails to achieve its intended outcome, and when these failures cannot be attributed to the intervention of some chance agency”. Human errors can be classified in two theoretical perspectives: the person approach and the system approach. Both the approaches have their own model of error causation and each model gives rise to different philosophies of error management.2.2.2 The person approach
The objective of the person approach system is to identify and classify the errors and violations made by the operator at the sharp end. The person approach views errors arise from unusual mental processes such as inattention, poor motivation, forgetfulness, carelessness, negligence, and recklessness (Reason, 2000). Researches related to the person’s approach try to classify the type and frequency of the errors made by an operator within complex systems, the ultimate aim being to recommend strategies, remedial measures and countermeasures designed to minimise future error occurrence (Salmon, Regan and Johnston, 2005). The person approach is a dominant tradition of blaming individual as it is easier to blame them for forgetfulness, inattention, or moral weakness rather than targeting institutes (Reason, 2000). Another major issue with the person approach system is that by focusing on the individual origins of error it isolates unsafe acts from their system’s context (Reason, 2000). From the number of person-based human error models developed and proposed, it can be concluded that there are many drawbacks associated with person-based error safety systems; due to the blame culture that they promote and the fact that successive errors are directed entirely at the individual and system wide failures are ignored to a large extent.2.2.3 The system approach
An alternative to the ‘person approach’ is the systems perspective approach, it identifies and treats an error as a systems failure, rather than an individual operator’s failure and considers the collective role of latent conditions and human errors in accident causation (Salmon, Regan and Johnston, 2005). Human error is not considered as a primary cause of incidents and accidents in system’s approach, instead it is seen as a consequence of the latent condition residing within the system (Salmon, Regan and Johnston, 2005). Reason (1990) proposed system perspective model of human error and accident causation and it is the most significant and widely recognised model available. The systems perspective model considers the interaction between latent conditions and errors and their contribution to organisational accidents. According to the model, a number of organisational levels contribute to the production of system outputs (e.g. decision makers, line management, productive activities and defences) (Salmon, Regan and Johnston, 2005). In an ideal world at each of the levels within the system, various defence layers exist. However in reality, they are like Swiss cheese slices (see Figure 4) with various holes. These holes can be taken as weaknesses in the defence created by latent conditions and errors, creating an opportunity for accidents (Salmon, Regan and Johnston, 2005; Reason, 2000). From all the system perspective-based error models available it can be concluded that the system based model of error proposed by Reason (1990) is the most popular and dominant model available and also the system based approach is the most appropriate approach for error management in the sociotechnical system.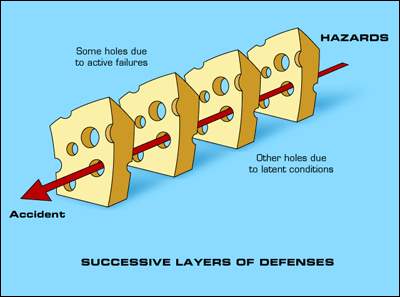
2.3 Accident Investigation and Analysis Methods
Unsafe acts and unsafe conditions contribute towards the majority of accidents. As it is not possible to identify and rectify all the hazards, the accident investigation programs are important for collecting critical data. The accident investigation is used to recreate accident situation and identify all the human and system based errors influencing an accident or incident scenario, to prevent future accidents. Sklet (2004) states that the accident investigation process is divided into three main phases by the Department of energy (DOE): the collection of data, the analysis of data and development of conclusions, and the development of findings. Numerous accident analysis models exist and each of the models has their own strengths and weaknesses and approach to the accident analysis. As stated in section 2.2.3 the system based accident analysis methods are applied to analyse and identify the complex circumstances and contributory factors within the accident. On discussion with the project supervisor some methods of great importance were selected for the analysis which were; Accimap accident analysis method, Human Factors Analysis and Classification System (HFACS), and Systems Theory Accident Modelling and Process (STAMP), and Event Analysis of Systemic Teamwork Framework (EAST; Salmon et al., 2011). Accimap is a generic accident analysis method used to represent and identify the key failures across six sociotechnical systems level. The Accimap approach does not have any classification or taxonomy of error types and it is used in various domains. The HFACS is a taxonomy based accident analysis method developed using Reason’s Swiss cheese model initially developed for aviation domain but later modified according to other domains requirement. STAMP is an accident analysis method used in various domains involving Mangatepopo gorge walking tragedy (Salmon, Cornelissen and Trotter, 2012) and Water Contamination Accident (Leveson et al., n.d.). It focuses on the control structure of the system in question and the failures involved at each level of control structure (Salmon et al., 2011). East is an accident analysis approach specifically developed for the analysis of collaborative activities. The method to date has been used for a number of different accident domains (Salmon et al., 2011). As mentioned in this section earlier Mangatepopo gorge walking tragedy occurred on 15th April 2008 to a group of 10 students and their teacher. The walking tragedy was analysed and compared using three systems accident analysis methods: Accimap, HFACS and STAMP. The outputs revealed significant differences on comparison among the three methods. On comparison, it was found out that Accimap and STAMP are most comprehensive among the three methods in terms of coverage of the overall sociotechnical system, but for single and complex accidents Accimap method was regarded as the most suitable method. However, for multiple accident case studies HFACS was considered as a more suitable method due to its taxonomic approach and a recommendation was made to add suitable taxonomies to the Accimap method so that it can be applied to multiple case study accidents (Salmon, Cornelissen and Trotter, 2012). Subsequently from the above four accident analysis methods final two methods were selected based on the facts that the methods are widely used in practice, well acknowledged and are described in the literature. Accimap and HFACS accident approach methods are chosen for analysis of the Hoegh Osaka grounding as both these methods are widely applied and have a different approach to the accident analysis. While, the Accimap accident analysis method is more domain independent as compared to HFACS, which is more aviation domain focused but due to its utility and flexibility it can be modified and applied for accident analysis purposes in wide range of domain. Whereas Accimap does not have any taxonomy or classification of errors hence it focuses on the casual flow of events upstream from the accidents on the other hand HFACS has taxonomies of failure modes at each of the four levels, errors and casual factors associated with the accidents can be classified by using the taxonomies. In Accimap failures are identified at each level and are linked between and across the levels, hence it is more of connections based method whereas HFACS is a more linear method as it does not have any links or connections amongst the four levels of taxonomies of failures or errors.3 Methodology
3.1 Methods chosen
From the discussion, in section 2.3 Accimap and HFACS accident analysis methods are chosen to analyse the grounding accident of Hoegh Osaka, even though both methods are systems method but they have a different approach for the analysis as mentioned in the section above.3.2 Accimap
3.2.1 Description of Accimap
Salmon et al. (2011:23) describes the Accimap method as “a system based accident analysis method that is specifically used to analyse and graphically represent the system based failures, decisions, and actions involved in an accident, occurring in socio-technical systems”. Originally developed by Rasmussen (1997) the method identifies environmental conditions and physical processes involved in an accident, it also identifies and represents the casual upstream flow of the events and the planning, management and regulatory bodies that may have contributed to the accident (Svedung and Rasmussen 2002). Six levels of complex sociotechnical frameworks are studied: Government Policy & Budgeting, Regulatory Bodies & Associations, Local Government & Company Management, Technical and Operational Management, Physical Processes & Actor Activities, and Equipment & Surroundings (See figure 5). Salmon et al. (2011) stated that these levels can be modified to suit the needs of the analysis. Moreover, failures identified at each level are linked between and across the levels based on cause-effect relations. As, Accimap is a generic approach it can be used for accident analysis purposes in any complex sociotechnical system (Salmon et al., 2011). The method has been applied to a range of accidents, involving Mangatepopo gorge walking tragedy (Salmon, Cornelissen and Trotter, 2012), police firearm accidents system (Salmon et al., 2011), and outdoor education system (Salmon et al., 2011).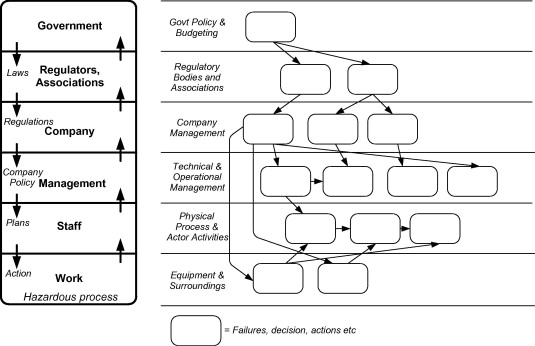
3.2.2 Procedure
The procedure is divided into six steps: data collection, construct an Actor Map, identify equipment and surroundings and physical actor activities failures, identify failures at other levels, construct draft Accimap diagram, and finalize and review Accimap Diagram. The following description is provided by Salmon et al. (2011).- Data Collection
- Construct an Actor map
- Identify equipment and surroundings and physical actor activities failures
- Identify failures at other levels
- Construct draft Accimap diagram
- Finalise and review Accimap diagram
3.2.3 Advantages and Disadvantages
Advantages Accimap approach identifies failures both at the sharp end and across the entire organisational level. It is simple to learn and use. Moreover, it permits identification of system failures and contributory factors at six different levels and it is a generic approach and has been used in various domains for accident analysis (Salmon et al., 2011). Disadvantages Accimap can be time consuming due to its size, and the quality of the analysis depends on the quality of input data. For complex accident scenarios, the analysis output can become large and inconvenient (Salmon et al., 2011).3.2.4 Reliability
Minimal or no reliability data of Accimap is present in the literature (Salmon et al., 2011). Reliability maybe low in some accident analysis as different analysts may describe events differently. Therefore, the quality of analysis depends exclusively on the analyst.3.3 Human Factors Analysis and Classification Systems (HFACS)
3.3.1 Description of HFACS
The Human Factors Analysis and Classification System (HFACS; Shappell and Wiegmann, 2000) was developed for use in the analysis of civil and military aviation accidents. It is based on Reason’s Swiss cheese model (SCM) (1990) and was subsequently developed using SCM as its core concept and an analysis of aviation accident report (Shappell and Wiegmann, 2000). It uses SCM’s four levels of human failure: unsafe acts, preconditions for unsafe acts, unsafe supervision, and organisational influences. The structure of the HFACS method is presented in Figure 6, which shows the different categories mapped onto Reason’s model.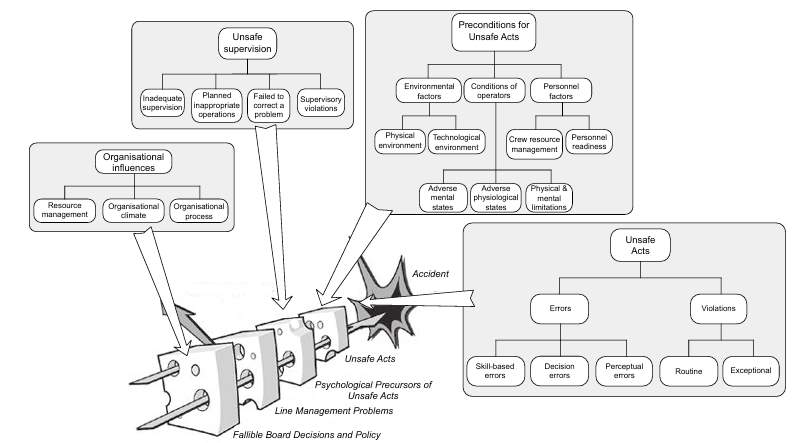
3.3.2 Description of Human Factors Analysis and classification Systems – Maritime Accidents (HFACS-MA)
HFACS-MA framework as shown in Figure 7 adopts the hierarchy of HFACS but some appropriate and necessary modifications have been made to level one (i.e. Unsafe Acts) and two (i.e. Preconditions) of HFACS model according to the requirements of International Maritime Organisation (IMO) guidelines and an additional level (i.e. External Factors) has been added on top of Organisational Influences. In the Preconditions level, it adheres to the SHEL model as the core to distinguish the types of causal factors (Chen et al., 2013).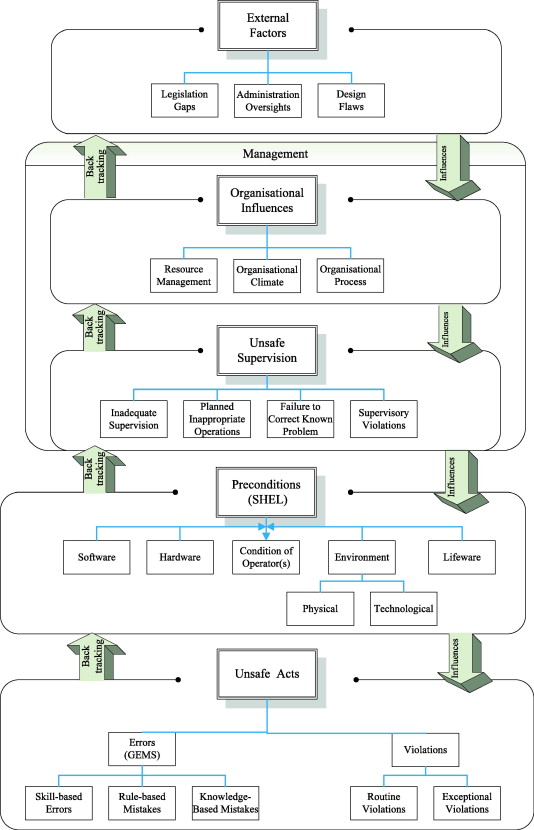
3.3.3 Procedure
The procedure is divided into eight steps: data collection, identifying unsafe acts involved, identifying failures at the pre-conditions for unsafe acts level, identify failures at the unsafe supervision level, identify failures at the organisational influences level, identify contributory external factors involved, review and refine the analysis. The following description is provided by Salmon et al. (2011).- Data collection
- Identifying unsafe acts involved
- Identifying failures at the pre-conditions for unsafe acts
- Identify failures at the unsafe supervision level
- Identify failures at the organisational influences level
- Identify contributory external factors involved
- Review and refine analysis
3.3.4 Advantages and Disadvantages
Advantages HFACS - MA offers an approach for identifying both the failures at the sharp end and those across the entire organisational system. It is simple to learn and the output is easily interpreted. It is based on Reason’s widely accepted and applied Swiss cheese model of organisational accidents, it also provides analysts with taxonomies of failures at five different levels and allows the associations between failures at the different levels to be analysed statistically (Salmon et al., 2011; Chen et al., 2013). Disadvantages It can be time consuming due to its comprehensiveness and the quality of the analysis depends on the quality of input data (Salmon et al. 2011).3.3.5 Reliability
A number of HFACS studies report inter-rater reliability statistics in terms of the level of agreement between different analysts using the approach to analyse the same incident (Salmon et al. 2011).3.4 Data collection and analysis
3.4.1 Data Collection
The Maritime Accident Investigation Branch (MAIB) investigation report of the Hoegh Osaka grounding is used as a primary source of data for the analysis.3.4.2 Incident synopsis
The Hoegh Osaka sailed for its journey to Bremerhaven on 3rd January 2006 with 24 crew members and a pilot on board. At 2109 the pure truck and car carrier developed a significant starboard while rounding West Bramble buoy in the Solent causing some cargo shift and consequent flooding. The ship lost steering and propulsion due to list in excess of 40 degree and subsequently drifted in the Bramble Bank grounding at 2115. Following the accident, the crew was successfully evacuated from the ship or recovered from the surrounding waters. Although during the accident majority of cargo remained in position, still 27% of the cargo sustained damage, varying from dents repairable scratches and to severe damage (MAIB Hoegh Osaka Grounding Accident Report, 2016).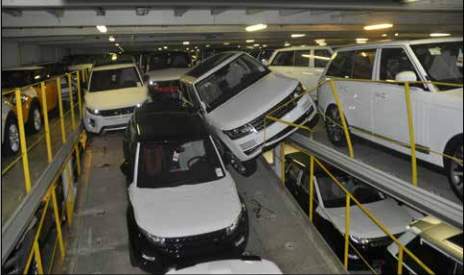
3.4.3 Data analysis
The Maritime Accident Investigation Branch (MAIB) report was used as the primary source of data in this project. It is analysed using two accident analyses methods to attempt to uncover all the possible factors accountable for the grounding of the ship.3.4.4 Reliability
The analysis of the Hoegh Osaka grounding has been reviewed by two Subject Matter Expert (SME). Both the SME’s specialise in the field of Accimap and HFACS and have been working or researching in the same field for few years. Both the analysis methods were reviewed and refined more than three times by the SME’s. Therefore, reliability of both the analysis should be of high level.4 Results of Hoegh Osaka ship grounding accident
4.1 The Accimap accident analysis method for the Hoegh Osaka grounding accident
The results of Hoegh Osaka grounding using Accimap is explained in detail in this section, which describes failures involved in the accident across six different levels. Various contributory factors are placed at relevant levels according to their features.4.1.1 Output and explanation of Actor map
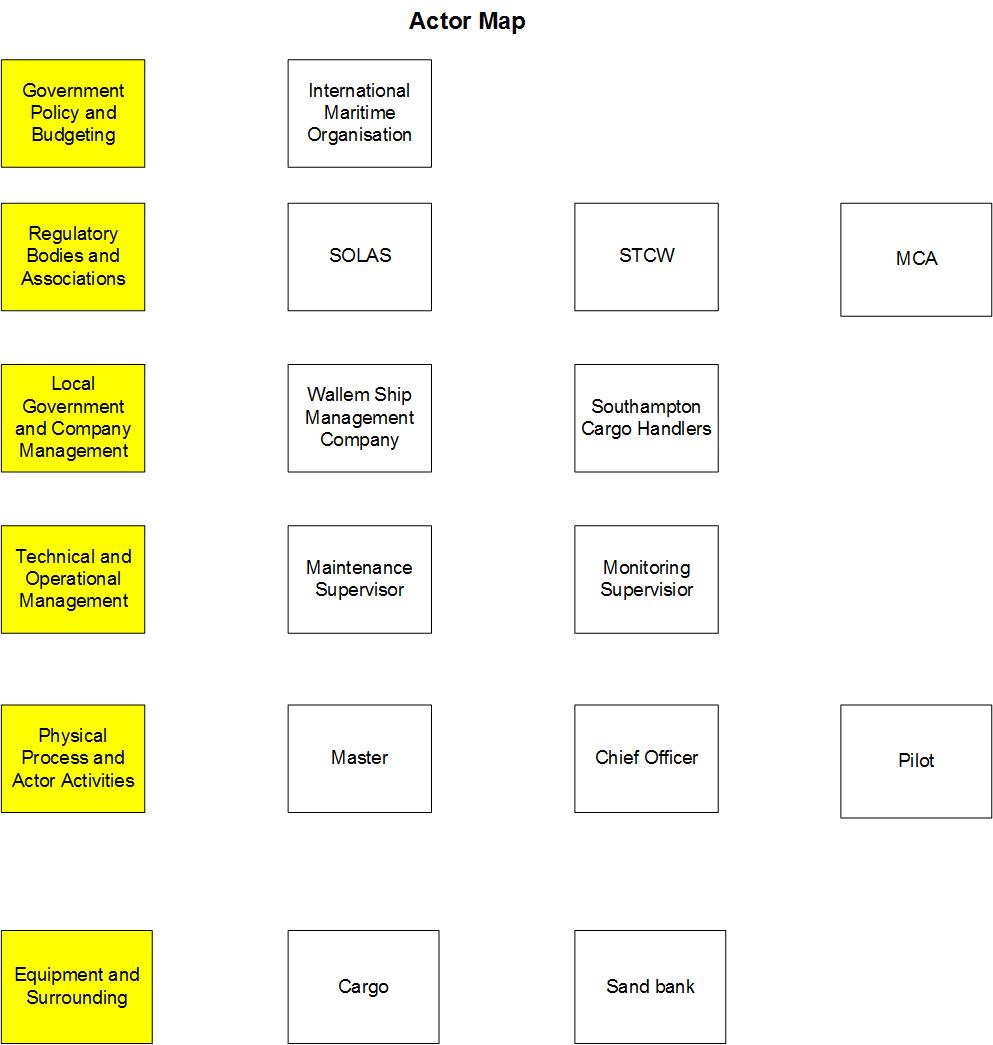
4.1.2 Output and explanation of Accimap
In figure 9 Accimap diagram has been produced, which represents all key failures involved at each of the six levels in the Hoegh Osaka grounding. A number of contributory factors are placed at one of the six levels and linked across given to their cause and consequence.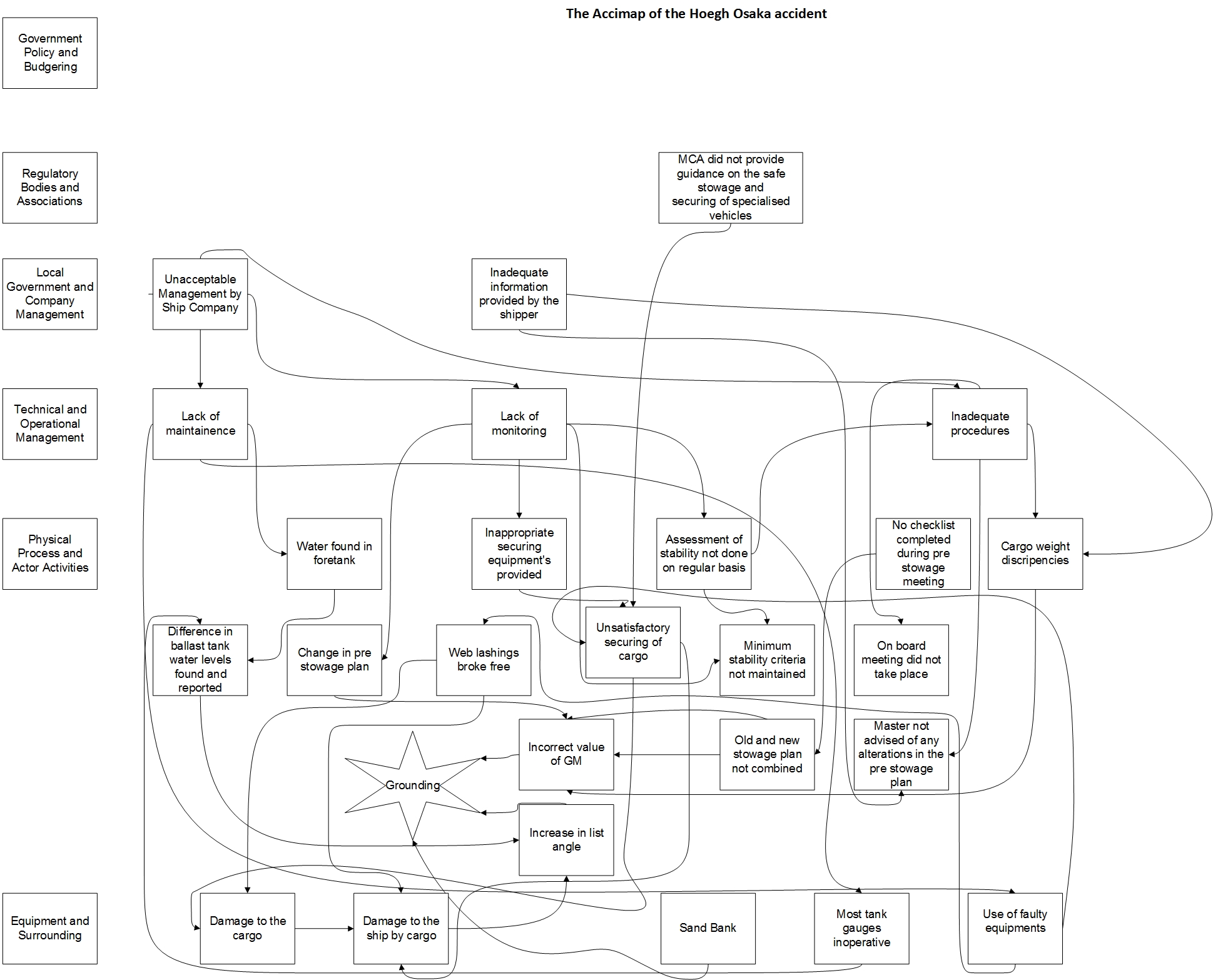
4.1.3 Government Policy and Budgeting
At the highest level, initially only one factor was identified as a key actor in the Actor map but during further analysis it was found that no factor was directly responsible for any failure at the level of government policy and budgeting.4.1.4 Regulatory Bodies and Associations
Three contributory factors including the Safety of Life at Sea Convection (SOLAS), the International Convention on Standards of Training, Certification and Watch keeping for Seafarers (STCW) and Maritime and Coastguard Agency (MCA) were identified at the regulatory bodies and associations level initially but as the analysis progressed no contributory factor from SOLAS and STWC was identified. The MCA does not have any regulation/policy to have all the booked cargo in port 24 hours prior to vessel loading as in Hamburg and Bremerhaven and no additional cargo is accepted during loading. Also, it did not provide any guidance regarding stowage and proper securing of the cargo.4.1.5 Local Government and Company Management
At this level, Wallem shipping company and Southampton cargo handlers were the two main failures identified related to the grounding. Wallem shipping company is Singapore based company which manages fleet in excess of 400 vessels of various types. In 2014 Wallem took over the technical management of the ship from Maersk. As a company, its duty is to follow and implement standard procedures to meet requirements of IMO and SOLAS. However, the company did not meet the IMO and SOLAS requirements which resulted in technical and operational management failures in the following level and SCH oversaw all the stevedoring operations on Hoegh Osaka, they had provided stevedoring services for many years and had a long running contract with them. The company did not inform the master or his representative in advance about the change in pre-stowage plan and it also failed to provide the right weight of the cargos being shipped which contributed to the failures in the following level. The company did not follow the SOLAS Chapter VI, Regulation 2, Paragraph 1 which states: “The shipper shall provide the master or his representative with appropriate information on the cargo sufficiently in advance of loading to enable the precautions which may be necessary for proper stowage and safe carriage to be put into effect” and Paragraph 3 states: “Prior to loading cargo units on board ships, the shipper shall ensure that the gross mass of such units is in accordance with the gross mass declared on the shipping documents” (MAIB Hoegh Osaka Grounding Accident Report, 2016).4.1.6 Technical and Operational Management
At the technical and operational management level, three contributory factors come from the above-mentioned level of local government and company management. The factors at this level contribute towards the failures at the physical process and actor activities level. As mentioned in the level above the Wallem shipping company failed to comply with the standards set by IMO and SOLAS for the shipping operations. Due to the lack of management, the company failed to technically maintain the ship which resulted in ship being operated with faulty gauges. As the ballast tank sounding could be taken manually it had not been considered as a priority by the company to repair the gauges which have been inoperative from the time of handover of the ship from Maersk. Moreover, the company failed to do regular checks regarding stability of ship and failed to provide proper securing equipments which lead to many failures in the last 2 levels.4.1.7 Physical process and Actor Activities
At the physical process and actor activities level, most of the failures identified occurred due to the factors at technical and management operational level. Wallem shipping company’s lack of maintenance and monitoring contributed towards maximum number of failures. As seen from the Accimap, chain of major failures occurred due to inoperative gauges due to which water was found in foretank that lead to the differences in water levels of ballast tanks found and reported. Due to the difference in water levels the list angle increased and assisted in the ship grounding. Moreover, due to the use of faulty and inappropriate securing equipments, it resulted in unsatisfactory securing of cargo which lead to web lashing breaking free. Also, no or minimal assessment of the stability of the ship was done which resulted in minimum stability criteria of the ship not being maintained. The master was not advised of any alteration in the stowage plan. Also, the chief officer failed to combine the new and old pre-stowage plans which lead to calculation of incorrect GM values. Moreover, no checklist was complete during pre-stowage meeting and as mentioned earlier SCH loaded additional cargos without informing the master, which resulted in pre-stowage plan being changed and the old and new stowage plan not being combined, which resulted in calculation of incorrect GM value.4.1.8 Equipment and Surrounding level
Various contributory factors are placed at the equipment and surrounding level. These include the equipment’s used (e.g. use of faulty web lashing and use of inoperative gauges) and environmental conditions (e.g. the Bramble bank turn). From five of the contributory failures identified, four failures occurred due to the failures at higher levels and as mentioned in section 4.1.7 web lashings used to secure the cargo broke free which damaged the cargo and the ship was damaged due to the cargo broke free which, resulted in water entering the ship compartments and hence increasing the list angle. It was also observed that the ship had the insufficient residual stability to survive the Bramble/sand bank turn at 12kt.4.2 HFACS-MA accident analysis method for the Hoegh Osaka grounding
The results of Hoegh Osaka grounding are explained in this section using HFACS-MA, which describes failures at five different heading using the taxonomy provided within the method.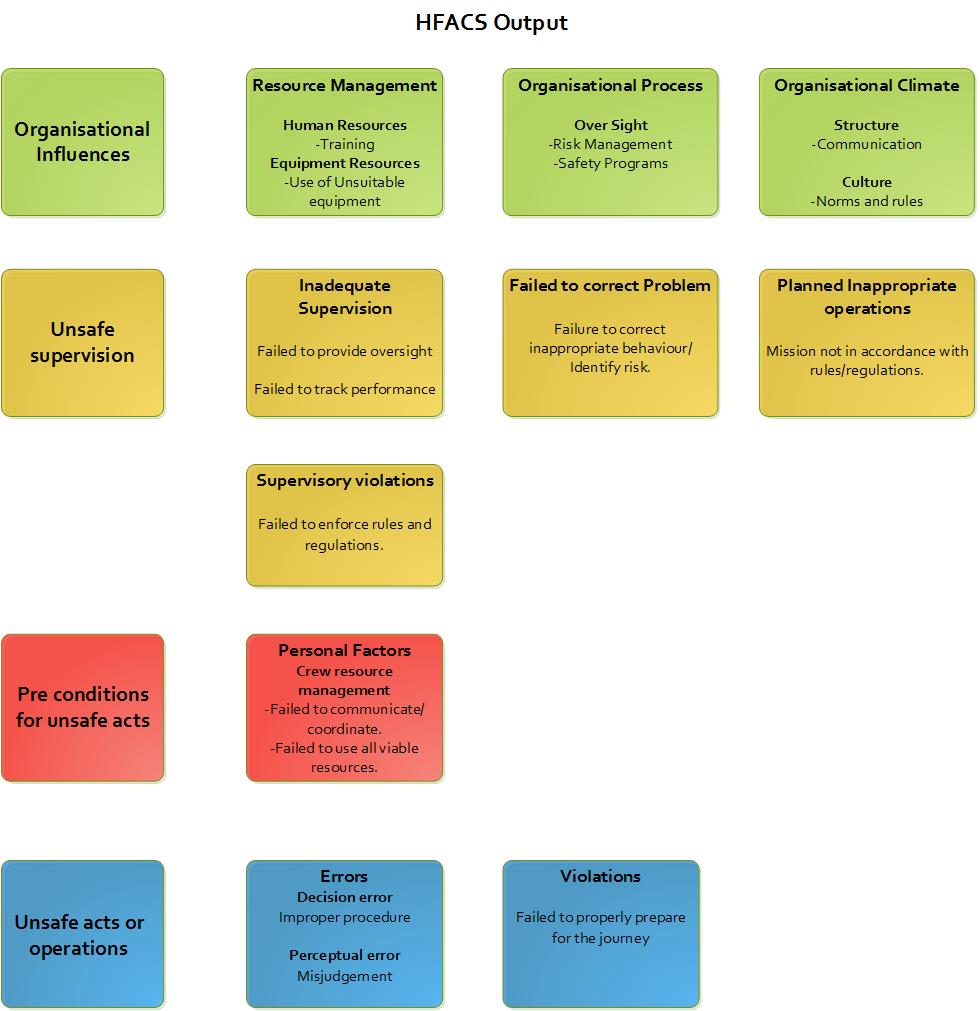
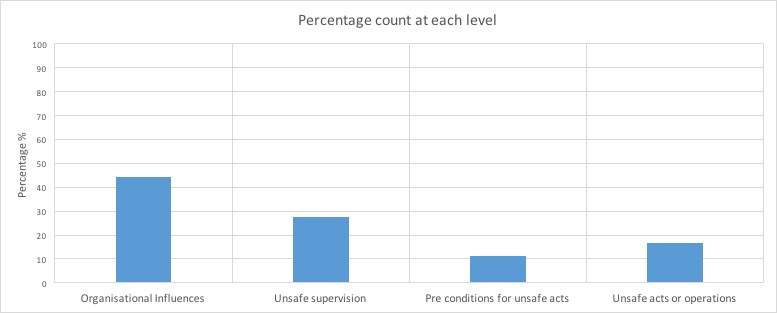
4.2.1 External Factors
There were no failures or contributory factors identified at the top most category.4.2.2 Organisational Influences
The organisational influences level describes the failures in the managing and running of the shipping company. In the ‘human resource’ category in the resource management category the training failure represents improper training procedures and similarly, from the resource management category, the sub category ‘equipment resources’ is represented through ‘use of unsuitable equipment’ failure which refers to use of improper equipment in the operation, which refers to use of faulty gauges due to which ballast tank soundings were made more difficult to record on daily basis and no clear highlights were made by the Hoegh auto liners cargo quality manual to either block-stow, or stow the cargo close to the ship’s side or in a position provided with sufficient securing points. Nearly one third of the failures at this level come from organisational process category. These occur in the ‘risk management and safety programs’ failure mode from the ‘oversight’ sub-category which represents inadequate risk management and safety system used by the Wallem ship management Pte limited and Hoegh autoliners. The Hoegh autoliners quality cargo manual and Wallem’s SMS are required to be modified and improved in number of ways. A similar number of failures as in organisational process category are identified in the organisational climate category. In the ‘structures’ sub-category the various communication failures are represented by the ‘communication’ failure mode, the Hoegh autoliners Cargo Quality Manual nor its internal cargo operations manual instructed the port captain to involve the chief officer in the preparation of the pre-stowage cargo plan and the Wallem PCC/PCTC Operations Manual provided no guidance on the role of the port captain, nor how the chief officer and port captain should co-operate to best effect. The final organisational climate failures fall under the ‘culture’ sub-heading. In the culture sub-heading, the routine violations allowed the by management are included in the ‘norms and rules’ failure mode which refers to the norm of not conducting a stability assessment of the ship until it has sailed.4.2.3 Unsafe Supervision
Various failures are classified at the unsafe supervision level. The Wallem shipping Pte’s and chief officer’s failures are represented through the ‘failed to provide oversight’ failure mode (i.e. failure to prioritise the repair of inoperative gauges by the Wallem shipping company and similar low priority was assumed by the chief officer who restored to estimating ballast tank quantities). Furthermore, the master and chief officer’s failures are represented under ‘failed to track performance’ (i.e. the master was advised of the ship’s stability criteria but he was unaware of how it was calculated), ‘failed to correct inappropriate behaviour/identify risk’ (i.e. the master did not identify that the ship had inadequate residual stability to survive the Bramble bank turn at 12kt), ‘failed to enforce rules and regulations’ (i.e. chief officers process of applying estimated figures to previously estimated figures caused him to assume a ballast condition for the ship’s departure which had no resemblance to the actual values) and ‘mission not in accordance with rules/regulations’ (i.e. on departure the ship had positive stability but its conditions did not comply with the IMO stability requirements).4.2.4 Preconditions for Unsafe Acts
Only two failures were classified at the pre-conditions for unsafe acts level. In the ‘personal factors’ category the failures ‘failed to communicate/coordinate’ and ‘failed to use all viable resources’ from the ‘crew resource management’ sub-category are represented. In the ‘failed to communicate/coordinate’ failure the port officer did not involve in any decision-making process with the chief officer or the master and in the ‘failed to use all the viable resources’ failure a LR approved loading computer program Loadstar was provided in the ship’s control centre to aid ship’s crew. The Loadstar manual contained an instruction to test the loading computer with standard conditions every 3 months and the last test file saved on board was for 31 December 2013.4.2.5 Unsafe Acts
At the unsafe acts or operations level, various errors and violations are identified which were committed by the chief officer. In the ‘errors’ category the failures ‘improper procedure’ and ‘misjudgement’ from the ‘decision error’ and ‘perceptual error’ sub-categories are represented. In the ‘improper procedure’ failure the chief officer did not follow the regular procedure required and underestimated the importance of accurately calculating the ship’s stability condition and in the ‘misjudgement’ failure the ship's ballast tank quantities were estimated by the chief officer and differed significantly from actual tank levels. In the ‘violations’ category the failure ‘failed to properly prepare for the journey’ is represented. In the ‘failed to properly prepare for the journey’ failure the chief officer did not calculate the stability condition accurately before the ship sailed as it had not been a cause of concern for him previously.5 Discussion
The aim of this dissertation is to compare the results using two contemporary accident analysis methods based on their application to analyses the Hoegh Osaka grounding accident. The comparison reveals key different finding between the methods in terms of methodology and output.5.1 Summary of result
During the analysis of the Hoegh Osaka grounding accident numerous human errors were identified using both Accimap and HFACS accident analysis method but these errors were considered as entire organisation’s errors instead of an individual’s error. Reason’s (1995) stated that accidents are caused by numerous factors covering human, organizational, environmental, technical elements, not only by a single unusual decision or action of a specific actor. For example, the chief officer was blamed for calculating wrong GM values of the ship whereas, it was due to the change in stowage plan due to which chief officer was not able to combine the old and new stowage plan in time. Moreover, another factor contributed towards the incorrect calculation of GM values, the chief officer was again held responsible for assuming wrong ballast tank values whereas the Wallem ship management company failed to repair the inoperative gauges which were inoperative since 2014 which forced the chief officer to take ballast tank soundings manually. Due to unsuitable procedures at higher levels, the chief officer calculated wrong GM values instead of correct values hence the reasons responsible for these human errors can be traced back to the higher levels in both the accident analysis methods.5.2 Comparison between Accimap and HFACS (HFACS-MA)
While using Accimap accident analysis method, analysts are given free reign to identify the contributing factors across the six levels placed. No taxonomy of errors or failure modes are given to help the analyst, and the entire system ranging from the role of government to the environmental conditions in which accident occurred are considered. All the contributory factors involved in an accident, ranging from government and local authority’s failures from many years before the incident to the operator failures on the day of the incident can be identified by using Accimap. Moreover, if the analyst is skilled enough and appropriate data is provided, the Accimap method can feasibly describe the entire accident path in terms of failures across the system and relationship between them. An important feature of Accimap is the linkage of failures within and between the six levels, this provides that the failures are considered in aspect of the failures affecting them. For example, in the present accident analyses the Accimap demonstrates that the chief officer’s decisions to assume the ballast tank quantities and not to conduct stability assessment until the ship has sailed were influenced by several factors across the system. The Accimap layout can be tricky/complex. For example, at the physical processes and actor activities level usually, faulty decisions are represented without necessarily identifying the factors influencing them. It can be problematic due to lack of taxonomy at each level as the analysis is fully dependent upon analyst’s independent judgement, hence the reliability of the method is expected to be limited. Different analysts are likely to identify and describe failures in different ways and may place them at different levels. Finally, due to the absence of taxonomies Accimap is considered more suitable to single case study analysis as compared to multiple case analyses Accident analysis methods like HFACS can be used to analyse multiple accident cases due to its taxonomic nature and HFACS reliability is enhanced as the analysts are given guidance in error and contributory factor classification. Its capability to associate failures across four levels is important as it allows the link between failures at four different levels to be assessed statically when used for multiple accident case analysis. This allows to identify the higher-level errors that influence the lower level operator errors and unsafe acts to be concentrated on for future accident prevention efforts. However, in HFACS accident analysis methods there are problems associated with the use of taxonomies. While the reliability of the analyses increases, the analyst is obliged in terms of specific failures that can be classified and when applying HFACS outside the aviation domain it becomes a problem as it was explicitly developed for aviation domain and numerous errors and failure modes are aviation specific (e.g. lack of aptitude to fly, misinterpretation of traffic calls) which makes them impracticable outside of the aviation domain. Furthermore, in HFACS analysis errors and contributory factors outside of the taxonomies cannot be categorised in the original method. In the present accident analysis, there were various failures that could not be classified, including the failure of the shipper to supply cargos with estimated weight instead of actual weight and failure by the chief officer as he failed to combine the new and old pre-stowage plan which lead to incorrect GM values. Moreover, with only four levels used in HFACS is tricky, as failures involving organisation (e.g. government policy, local authority level failures) are not considered. However, recent HFACS analyses have used additional ‘outside factors’ levels which include ‘regulatory factors’ and ‘other’ failure categories e.g. Chen et al., (2013).5.3 Recommendations
Recommendations from the results of Accimap and HFACS accident analysis methods are presented below to prevent future accidents. The proposed recommendations across six levels of Accimap to countermeasure the corresponding systematic and human errors are presented below. Government policy and budgeting: -- IMO should issue a statement or a warning in context to the Hoegh Osaka grounding accident to notify all its members regarding the importance of accurately calculating the stability of the ship after loading to ensure ship’s safe voyage.
- MCA should provide guidance on safe stowage and securing of special vehicles.
- It should also make a rule to have all the booked cargo in port at least 24 hours prior to vessel loading and not allow additional cargo to be loaded on the ship as in Germany.
- SCH are advised to provide actual cargo weight when available rather than estimated weight while preparing a ship’s final cargo tally.
- Wallem ship management Pte limited are advised to make their PCC/PTCT training course compulsory.
- Wallem ship management Pte limited should repair and test all the faulty gauges before the vessel is brought into use again.
- It should carry ship maintenance checks at regular intervals.
- It should also provide appropriate and sound equipment for stowage purposes.
- It should issue a notice to all its masters and crew members regarding the importance of following all the procedures mentioned in its PCC/PTCT manual.
- All masters and the crew members are advised to carry out all necessary procedures and steps prior to and on the voyage irrespective of any perceived commercial pressure.
- Regular checks should be made to ensure all the equipments are in sound condition.
- Weather conditions should be checked prior to departure and measures should be taken accordingly if necessary.
- Wallem ship management Pte Ltd. are advised to include the stability calculation method of the ship in their 2-day training course and they are advised to make the training course compulsory for all the newly assigned officers.
- Hoegh autoliners shipping Pte are recommended to update their cargo manual on how to properly secure cargo.
- The organisation is advised not to approve any itinerary changed due to commercial pressure.
- Both Wallem and Hoegh autoliners are advised to modify and update their respective SMS’s and quality cargo manual.
- Both the companies are advised to draw guidelines regarding communication between the master and the other crew members.
- The companies are recommended to carry out surprise checks to ensure that all the required procedures are followed by the master and the crew members.
- The Wallem ship management Pte ltd is advised to repair and test all the faulty gauges before the vessel is brought into use again.
- The master is advised to track and follow every step used in calculating the ship’s stability assessment.
- The master and the chief officer are advised to comply with all the stability requirements required by the IMO before and after departure.
- The chief officer is advised to use all the available resources or equipment available to calculate the ship’s stability.
- The port captain and chief officer are advised to take part in decision making regarding the ship wherever possible.
- The chief officer is advised to follow all the necessary procedures required to calculate the ship’s stability before its departure.
5.4 Evaluation
The Hoegh Osaka grounding accident is a complex accident, hence there might be some imperfections in the analysis even though as mentioned in section 3.4.4 the analysis has been updated and modified several times by two SME’s for both Accimap and HFACS accident analysis methods, still there might be some imperfections in the analysis as identifying and linking of failures across six level of Accimap changes among different analyst. Similarly, for HFACS even though taxonomies are provided for each failure mode different analysts may place failures at different levels.5.5 Future research
Due to the limited time, the analysis was only reviewed by the SME’s and no peer reliability assessment was done for both the methods. For future works, peer reliability assessment can be done to increase the reliability of the analysis. For the peer reliability assessment, at least 6-8 colleagues or course mates can be invited to take part who were not part of the dissertation and they can be explained in brief about the accident and both the accident analysis methods. Next, they can be asked to place and link all the errors accordingly in both the methods where applicable and then their analysis can be compared with the original analysis, if the percentage score of agreement is above 75%, then the analysis can be deemed reliable.6 Conclusion
In this dissertation, two completely different types of accident analysis methods were used to analyse the Hoegh Osaka grounding accident to compare the results obtained. Systems errors were identified through both Accimap and HFACS, therefore the whole grounding accident cannot be blamed on an individual. As in Accimap at regulatory bodies and association level, SCH did not have any regulation or policy in Southampton to have all the booked cargo in port 24 hours prior to vessel loading. At local government and company management, the Wallem ship Management Company and SCH failed to meet the IMO and SOLAS requirements. At the technical and operational management level, maintenance and monitoring procedures were not done on regular basis. In addition, at the equipment and surroundings level, use of inappropriate equipment and natural conditions like Bramble bank along with all systematic failures considered together influenced the human errors in the Hoegh Osaka grounding accident. Similarly, in HFACS most of the human errors were influenced by higher 2 categories. As seen from figure 12 it can be said that a maximum number of systematic failures occurred at the top most category and it may have induced the errors at lower categories, resulting in the grounding accident. Moreover, the Accimap accident analysis method has been considered as the more suitable method for detailed analysis of single and complicated accidents from the two methods. As in Accimap, the analyst is not confined by taxonomies of failure modes hence making it comprehensive and straightforward to use as compared to HFACS. However, for multiple accident case studies HFACS being a taxonomical approach is more useful and it is likely to be more reliable as compared to Accimap. But the disadvantage of HFACS is that it does not have domain specific taxonomies and taxonomies considering higher government and local authority failures. Hence, the analysts may not be able to classify all the failures involved and the contributory factors maybe missed while applying the method in any domain other than aviation.7 References
Anon, (2012). International Shipping Facts and Figures – Information Resources on Trade, Safety, Security, Environment. [Online] Available at: http://www.imo.org/en/KnowledgeCentre/ShipsAndShippingFactsAndFigures/TheRoleandImportanceofInternationalShipping/Documents/International%20Shipping%20-%20Facts%20and%20Figures.pdf [Accessed 22 Mar. 2017]. Anon, (2016). [Online] Available at: http://www.agcs.allianz.com/assets/PDFs/Reports/AGCS_Safety_Shipping_Review_2016.pdf [Accessed 28 Mar. 2017]. Chauvin, C., Lardjane, S., Morel, G., Clostermann, J. and Langard, B. (2013). Human and organisational factors in maritime accidents: Analysis of collisions at sea using the HFACS. Accident Analysis & Prevention, 59, pp.26-37. Chen, S., Wall, A., Davies, P., Yang, Z., Wang, J. and Chou, Y. (2013). A Human and Organisational Factors (HOFs) analysis method for marine casualties using HFACS-Maritime Accidents (HFACS-MA). Safety Science, [online] 60, pp.105-114. Available at: http://www.sciencedirect.com/science/article/pii/S0925753513001380 [Accessed 17 Feb. 2017]. Emsa.europa.eu. (2017). Latest News - Annual Overview of Marine Casualties and Incidents 2016 - EMSA - European Maritime Safety Agency. [Online] Available at: http://www.emsa.europa.eu/news-a-press-centre/external-news/item/2903-annual-overview-of-marine-casualties-and-incidents-2016.html [Accessed 28 Mar. 2017]. Leveson, N., Daouk, M., Dulac, N. and Marais, K. (n.d.). Applying STAMP in Accident Analysis. 1st ed. [ebook] Available at: https://shemesh.larc.nasa.gov/iria03/p13-leveson.pdf [Accessed 18 Apr. 2017]. LUO, M. and SHIN, S. (2016). Half-century research developments in maritime accidents: Future directions. Accident Analysis & Prevention. [Online] Available at: http://www.sciencedirect.com/science/article/pii/S0001457516301178 [Accessed 21 Mar. 2017]. MAIB Hoegh Osaka Grounding Accident Report. (2016). 1st ed. [eBook] Available at: https://assets.publishing.service.gov.uk/media/56e9a7afe5274a14d9000000/MAIBInvReport6_2016.pdf [Accessed 18 Oct. 2016]. Martins, M. and Maturana, M. (2010). Human Error Contribution in Collision and Grounding of Oil Tankers. Open Minds! (Formerly: Moving Images blog). (2012). Remembering Asia’s Titanic: The Doña Paz tragedy that killed over 4,000 in Dec 1987. [Online] Available at: https://nalakagunawardene.com/2012/04/13/remembering-asias-titanic-the-dona-paz-tragedy-that-killed-over-4000-in-dec-1987/ [Accessed 28 Mar. 2017]. Rasmussen, J. (1997). Risk management in a dynamic society: A modelling problem. 1st ed. [eBook] Available at: http://sunnyday.mit.edu/16.863/rasmussen-safetyscience.pdf [Accessed 17 Mar. 2017]. Reason, J. (1990). Human error. 1st ed. Cambridge [England]: Cambridge University Press. Reason, J. (2000). Human error: models and management. BMJ, [online] 320(7237), pp.768-770. Available at: http://www.bmj.com/content/bmj/320/7237/768.full.pdf [Accessed 26 Mar. 2017]. Rothblum, D. (2002). HUMAN FACTORS IN INCIDENT INVESTIGATION AND ANALYSIS. [Online] Available at: http://citeseerx.ist.psu.edu/viewdoc/download?doi=10.1.1.840.8053&rep=rep1&type=pdf [Accessed 15 Mar. 2017]. Salmon, P., Regan, M. and Johnston, I. (2005). HUMAN ERROR AND ROAD TRANSPORT. 1st ed. [eBook] Available at: https://www.monash.edu/__data/assets/pdf_file/0010/216946/muarc256.pdf [Accessed 27 Mar. 2017]. Salmon, P., A. Stanton, N., P. Jenkins, D., Rafferty, L. and H. Walker, G. (2011). Human Factors Methods and Accident Analysis: Practical Guidance and Case Study Applications. 1st ed. Ashgate Publishing Group. Salmon, P., Cornelissen, M. and Trotter, M. (2012). Systems-based accident analysis methods: A comparison of Accimap, HFACS, and STAMP. Safety Science, [online] 50(4), pp.1158-1170. Available at: http://www.sciencedirect.com/science/article/pii/S0925753511002992 [Accessed 12 Nov. 2016]. Shappell, S. and Wiegmann, D. (2000). The Human Factors Analysis and Classification System–HFACS. [Online] Available at: https://www.nifc.gov/fireInfo/fireInfo_documents/humanfactors_classAnly.pdf [Accessed 15 Dec. 2016]. Sklet, S. (2004). Comparison of some selected methods for accident investigation. Journal of Hazardous Materials, [online] 111(1-3), pp.29-37. Available at: https://dvikan.no/ntnustudentserver/reports/Comparison%20of%20some%20selected%20methods%20for%20accident%20investigation.pdf [Accessed 28 Mar. 2017]. Svedung, I. and Rasmussen, J. (2002). Graphic representation of accident scenarios: mapping system structure and the causation of accidents. Safety Science, [online] 40(5), pp.397-417. Available at: http://www.sciencedirect.com/science/article/pii/S0925753500000369 [Accessed 10 Mar. 2017]. The Lusitania Resource. (2016). the Lusitania Resource. [Online] Available at: http://www.rmslusitania.info/ [Accessed 28 Mar. 2017]. Time.com. (2016). The Forgotten Maritime Tragedy Deadlier Than the Titanic. [Online] Available at: http://time.com/4198914/wilhelm-gustloff-salt-to-the-sea/ [Accessed 28 Mar. 2017]. Titanic, D. and sinks, T. (n.d.). Titanic sinks - Apr 15, 1912 - HISTORY.com. [Online] HISTORY.com. Available at: http://www.history.com/this-day-in-history/titanic-sinks [Accessed 28 Mar. 2017].Cite This Work
To export a reference to this article please select a referencing stye below:
Related Services
View allRelated Content
All TagsContent relating to: "Transportation"
Transportation refers to the process of goods, people or animals being moved from one place to another. There are multiple methods of transport, including train, boat, bus, plane, lorry, and more.
Related Articles
DMCA / Removal Request
If you are the original writer of this dissertation and no longer wish to have your work published on the UKDiss.com website then please: